the Creative Commons Attribution 4.0 License.
the Creative Commons Attribution 4.0 License.
In vitro rumen fermentation pattern: insights from concentrate level and plant oil supplement
Shahryar Kargar
Golnaz Taasoli
Amir Akhlaghi
Mohammad Javad Zamiri
The addition of oil to ruminant diets and oil fatty acid profiles are major factors that negatively affect ruminal fermentation, while increased forage level attenuates the adverse effects. The aim of this study was to determine the effects of oil source supplementation and concentrate level in the diet on in vitro ruminal fermentation kinetics. Pomegranate, garlic or sunflower oils were added (2 % dry matter (DM) basis) to the diets containing 40 % or 60 % (DM basis) concentrates. In vitro gas production parameters, pH, ammonia nitrogen concentration and total protozoa count were measured. Additionally, metabolizable energy (ME), short-chain fatty acid (SCFA) production and organic matter digestibility (OMD) were determined. Rumen fermentation parameters and protozoal population counts were analyzed as a completely randomized design with a 2 × 3 factorial arrangement of treatments, and gas production parameters were analyzed as a 2 × 3 factorial arrangement in a randomized block design. The results showed that the HCPO (high (60 %) concentrate diet containing pomegranate oil) and HCSO (high (60 %) concentrate diet containing sunflower oil) diets produced the highest (5.40 mg dL−1) and lowest (2.61 mg dL−1) concentrations of NH3–N (p>0.01), respectively. Total protozoa count tended (p=0.07) to be highest in HCPO and lowest in HCSO diets (5.10 vs. 4.81 Log 10 g−1 digesta). No interaction effects between the concentrate level and oil source were found on in vitro gas production parameters, pH, estimated ME, SCFA and OMD, and Entodinium and Diplodinium populations (p>0.05). It is concluded that dietary supplementation with highly unsaturated oil from three different sources at 2 % level (DM basis) had no apparent effects on in vitro ruminal fermentation patterns.
- Article
(430 KB) - Full-text XML
- BibTeX
- EndNote
Feeds supplemented with oils rich in polyunsaturated fatty acid (PUFA) were extensively investigated in the last decades. Generally, oil supplements exert toxic effects on the gram-positive bacteria and ciliate protozoa (Nagaraja et al., 2007) and limit the microbial colonization of feed particles and the access of microbial enzymes to the substrates (Jenkins, 1993), but they have an ability to change the rumen fatty acid proportions positively by affecting the activity of rumen microorganisms (Abu Ghazaleh and Ishlak, 2014). For example, c9t11 CLA is the most abundant isomer of conjugated linoleic acid (CLA) in ruminant-derived foods and most of this isomer is produced in animal tissues via D9 desaturase using vaccenic acid (VA, t11 C18:1) as its substrate (Griinari et al., 2000). Therefore, increasing the formation and flow of VA from the rumen is desirable as it would aid in the process of developing healthier ruminant-derived food products. Supplement ruminant animal diets with plant oils (Váradyová et al., 2007; Doreau et al., 2009) resulted in an increase in the formation and flow of VA from the rumen.
There has been increased interest in the effect of unconventional oil sources, such as pomegranate and garlic oil, on ruminal fermentation. Conjugated octadecatrienoic fatty acids account for about 80 % of the pomegranate seed fatty acids. Punicic acid (cis9, trans11, cis13 acid) is the most ubiquitous (31 %–86 %) fatty acid in pomegranate oil, followed by linoleic acid (0.7 %–24.4 %) and oleic acid (0.4 %–17.4 %) (Khoddami and Roberts, 2015). A study on four cultivars of Iranian pomegranate seed oil showed that 91.8 %–92.1 % of the fatty acids were unsaturated (Dadashi et al., 2013). However, the oil content of the seeds and fatty acid composition are affected by cultivation sites, harvesting time, fruit genotypes and climatic conditions (Fadavi et al., 2006). Garlic (Allium sativa) is a member of Alliaceae or Liliaceae family (Sethi et al., 2014). Linoleic (53.6 % of FA content) and palmitic acids (20 % of FA content) form a large proportion of fatty acids in garlic oil (Tsiaganis et al., 2006).
The fatty acid profiles of pomegranate and garlic oil differ from those of the more conventional plant oil; consequently, their impact on ruminal microorganisms and fermentation pattern may be different. However, this has been little researched.
Rumen fermentation is affected by the concentrate-to-forage ratio (Chen et al., 2021), and the effect of unsaturated fatty acids on ruminal function may be different between the diets containing different levels of concentrates (Ueda et al., 2003). High-concentrate diets generally decrease the ruminal fluid pH; therefore, rumen microbes and their fermentation product are probably different in comparison to low-concentrate diet (Saliba et al., 2014). Hence, it is necessary to address the interactive effects of PUFA-rich oils and diet on ruminal fermentation pattern and microbial populations. Therefore, the objective of this study was to compare the effect of pomegranate and garlic oil with sunflower oil (as a conventional oil source) in diets containing different forage : concentrate ratios using an in vitro gas production system. The hypothesis was that high PUFA content of the pomegranate and garlic oil would change the ruminal fermentation kinetics.
2.1 Experimental diets
The ingredients and composition of the experimental diets are presented in Table 1. The diets were formulated according to NRC recommendations (NRC, 2001). Dietary samples were dried at 60 ∘C in a forced-air oven for 48 h and milled through a 2.0 mm screen using a laboratory mill (Thomas Scientific, Swedesboro, NJ, USA). Sunflower, pomegranate or garlic oil was added (20 g kg−1 dry matter (DM)) to either high- or low-concentrate basal diets (Table 1). Fatty acid compositions of the oil sources are presented in Table 2. The profile of fatty acid methyl esters (FAME) was determined by gas chromatography (GC; Agilent Technologies 7890 AGC System (Agilent Technologies, Inc., USA) equipped with a flame ionization detector). Samples were injected by split injection (split ratio 1:50). The carrier gas was hydrogen, inlet pressure 246.38 kPa. Fatty-acid peaks were identified based on their retention times. Separation of FAME was realized with a Supelco column (SP-2560, Sigma-Aldrich) (75 m × 180 µm × 0.14 µm). The temperature program ran from a starting temperature of 70 ∘C for 2 min, increasing 15 ∘C min−1 to 150 ∘C; from 150 to 165 ∘C, an increase of 1 ∘C min−1 was established. Then, 165 ∘C was held for 12 min; from 165 to 170 ∘C, the temperature increased 2 ∘C min−1 with 170 ∘C held for 5 min and from 170 to 215 ∘C, an increase of 5 ∘C min−1 was applied, with the final temperature kept for 10 min. Fatty acid methyl esters were determined with Agilent ChemStation software (B.04.03, Agilent Technologies, Inc., USA; Edition 9/2010), and tridecanoic acid (C13:0) (as triacylglyceride; Sigma, Bornem, Belgium) was used as internal standard.
Table 1Ingredients and chemical composition of the experimental diets.
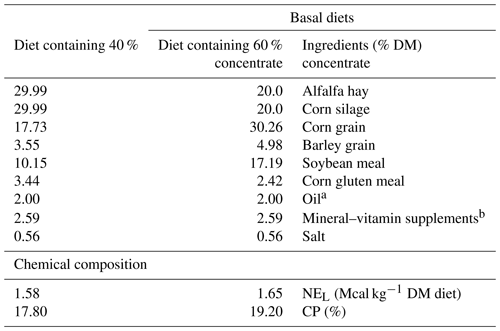
a Pomegranate, garlic or sunflower oil added to the incubation medium. b Mineral and vitamin premix contained 50 g kg−1 of Ca; 32 g kg−1 of P; 11 g kg−1 Mg; 2 g kg−1 Mn; 3 g kg−1 Fe; 2 g kg−1 Zn; 1 g kg−1 Cu; 60 mg kg−1 I; 60 mg kg−1 Co; 2 mg kg−1 Se; 250 000 IU kg−1 vitamin A; 100 000 IU kg−1 vitamin D3; 100 IU kg−1 vitamin E; 20 mg kg−1 vitamin B1 and 40 mg kg−1 B2.
2.2 In vitro gas production measurement
In vitro gas production was run in duplicate, using the rumen fluid collected from two rumen-fistulated Kurdish rams (65 ± 4 kg body weight) fed a diet containing 40 % alfalfa and 60 % concentrates (DM basis). Rumen fluid samples were collected prior to the morning feeding, to reduce variability between incubations (Muetzel et al., 2014), and transported to the laboratory in prewarmed insulated flasks. The contents were squeezed through a four-layer cheesecloth and then mixed with prewarmed buffer, at a ratio of 1:2 (fluid to buffer) under continuous CO2 flushing (McDougall, 1948). Gas production was measured as described by Theodorou et al. (1996). Feed samples were ground to pass a 1 mm screen. Approximately 250 mg of each experimental diet was transferred into tubes kept at 39 ∘C and flushed with CO2 before use. Oils were added to the tubes at “5 µL/250 mg diet”. Each sample was incubated in triplicate. After equilibrating the solution with CO2, a 40 mL aliquot of rumen fluid–buffer mixture was added to 125 mL incubation bottles containing 250 mg of the experimental diets. Rumen fluid–buffer mixture was anaerobically dispensed in each bottle at 39 ∘C. The bottles were crimped, placed in an incubator at 39 ∘C, and shaken at regular intervals (every hour). Three bottles containing buffered rumen fluid only were used as blank samples. The pressure of gas produced in each bottle was recorded using a pressure transducer (Manometer Digital testo 512; Testo, Lenzkirch, Germany) at 2, 4, 6, 8, 12, 18, 24, 48, 72 and 96 h following the initiation of incubation. To estimate the kinetics of gas production, the data on cumulative gas volume produced were fitted to an exponential model () in which p represents the in vitro gas production (mL) at time t, (a+b) is the potential gas production, and c the fractional rate of gas production per hour. The model allowed for the estimation of a lag phase (lag) before rapid gas production began (McDonald, 1981).
The half-life (, h) of the degradable fraction of each substrate was calculated as the time taken for gas accumulation to reach 50 % of its asymptotic.
The volume of gas produced (GP) after 24 h of incubation and crude protein (CP) and crude fat contents were used to estimate the metabolizable energy (ME) concentration (MJ kg−1 DM) and organic matter digestibility (OMD) based on the following equations (Menke and Steingass, 1988):
where GP is gas production after 24 h, XP is crude protein (%), XL is crude fat (%) and XA is ash (%).
Short-chain fatty acid (SCFA) production (mmol/200 mg DM) was calculated from the following equation (Makkar, 2010):
2.3 Ammonia nitrogen (NH3–N) and pH measurement
Ammonia nitrogen and pH (pH meter 330i, WTW GmbH, Weilheim, Germany) in the incubation media were measured after 24 h incubation. For NH3–N measurement, a 4 mL sample of the rumen fluid–buffer mixture in each bottle was acidified with 4 mL of 0.2 N HCl and stored at −20 ∘C pending further analyses. Ammonia nitrogen was determined according to the procedure described previously (Broderik and Kang, 1980). Briefly, the sample was thawed, shaken and centrifuged at 2500 × gfor 20 min, and the supernatant was read on a spectrophotometer (UV-120-01; Shimadzu, Kyoto, Japan) at 630 nm.
2.4 Rumen protozoal population and genera distribution
Incubation was stopped after 24 h, and the inoculants were subjected to protozoal enumeration. Rumen ciliates were identified according to the method of Dehority (2003). Two milliliters of rumen fluid–buffer mixture in each bottle were pipetted into a screw-capped test tube containing 5 mL of formalin–normal saline solution (20 mL formalin in 100 mL normal saline). Two drops of brilliant green dye (2 g brilliant green and 2 mL glacial acetic diluted in 100 mL distilled water) were then added to the test tube, mixed thoroughly and allowed to stay overnight at room temperature. Total and differential counts of protozoa were made in 30 microscopic fields at a magnification of 100× on a counting slide using five replicates. The position of ciliary zone and size of the cell were considered differential characteristics for distribution of the genera. To conform to normal distribution, the protozoal counts were transformed to log10 basis prior to statistical analysis.
2.5 Statistical analysis
All statistical analyses were performed using the PROC MIXED of the SAS software (SAS 9.4). Rumen fermentation parameters and protozoal population counts were analyzed as a completely randomized design with a 2 × 3 factorial arrangement of treatments. The model included the fixed effects of oil source, concentrate level and oil source × concentrate level. Gas production parameters were analyzed as a 2 × 3 factorial arrangement in a randomized block design. Treatment means were compared using Tukey's multiple range tests if the interaction effect was not significant.
3.1 In vitro gas production parameters, ammonia nitrogen (NH3–N) concentration and pH
There were no significant effects of the dietary concentrate level, oil source and their interaction (p>0.05) on gas production potential, gas production rate and lag time (Table 3). The estimated metabolizable energy (ME), short-chain fatty acid (SCFA) production and organic matter digestibility (OMD) tended to be different among oil sources (p=0.07), but not affected by the dietary concentrate level or its interaction with the oil source (p>0.05; Table 3).
The ruminal fluid pH was not affected by any dietary combinations (Table 3). The oil source did not influence in vitro ruminal fluid NH3–N concentration in the diet containing 40 % concentrates (Table 3); however, in the diet containing 60 % concentrates, the highest and lowest concentrations of NH3–N were produced in the presence of pomegranate (5.40 mg dL−1) and sunflower (2.61 mg dL−1) oil, respectively, with intermediate values recorded for garlic oil (4.57 mg dL−1).
Table 3The effect of sunflower, pomegranate and garlic oil on gas production characteristics, and ruminal pH and ammonia nitrogen concentration in diets containing 40 % or 60 % concentrates.
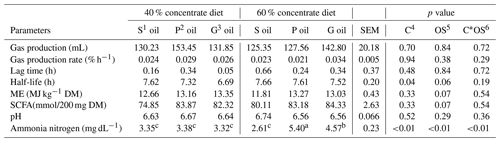
1 Sunflower oil. 2 Pomegranate oil. 3 Garlic oil. 4 Concentrate level. 5 Oil source. 6 Concentrate level by oil source interaction. a,b Means within a row with different superscripts are significantly different (P≤0.05).
3.2 Rumen protozoal population and the genera distribution
The total protozoal count (p=0.07) and Ophrioscolex population (p=0.08) tended to be influenced by the interaction effect of the concentrate level and oil source (Table 4). Furthermore, in 60 % concentrate diet, the highest total protozoa count (5.10 Log10 g−1 digesta) was recorded for pomegranate oil and the lowest number (4.81 Log10 g−1 digesta) for sunflower oil.
Table 4The effect of sunflower, pomegranate and garlic oil on protozoal counts in diets containing 40 % or 60 % concentrates.
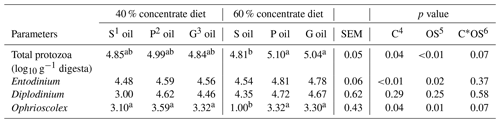
1 Sunflower oil. 2 Pomegranate oil. 3 Garlic oil. 4 Concentrate level. 5 Oil source. 6 Concentrate level by oil source interaction. a,b Means within a row with different superscripts are significantly different (P≤0.05).
4.1 In vitro gas production
Gas production is mainly due to fermentation of carbohydrates to acetate, propionate and butyrate, with a high-correlation short-chain fatty acid and gas production (Blümmel and Orskov, 1993). The lack of effect of the oil source on gas production and lag time may be due to the fact that oils could not decrease microbial colonization and consequently digestion of diets. These results are in line with the studies reporting little or no effect of low level of oil supplementation (Patra, 2013; El-Sherbiny et al., 2016; Roy et al., 2017). On the other hand, addition of 5 or 10 mg pomegranate oil to alfalfa hay and concentrate reduced total in vitro gas production (Maleki et al., 2016), and oleic acid (35 and 70 g kg−1 of substrate dry matter) decreased in vitro gas production in the diet containing rye and cornmeal (Zhang et al., 2008). This decrease could be associated with the suppression of density and activity of rumen bacteria by dietary UFA supplementation, particularly the cellulose-utilizing bacteria (Palmquist and Jenkins, 1980). The addition of 2 % sunflower oil to rumen simulation technique (RUSITEC) containing starch increased the lag time in comparison with the control diet or cellulose (Vargas et al., 2017). Collectively, the conflicting data on in vitro gas production experiments could be due to use of different substrates, dosage of supplemental long-chain fatty acids, and associated rumen microbes (Wu et al., 2016).
4.2 In vitro fermentation
In the present study, pH values were within normal ranges (6–7). The oil source, FA composition, and supplementation dose could affect the ruminal pH. High-dose vegetable oil supplementation, providing high levels of PUFA, changes the ruminal pH and the fermentation pattern (Ivan et al., 2001). In line with the current findings, supplementation of 6 % olive oil or sunflower oil and/or linseed oil to the diets containing 70 % concentrate (Vargas et al., 2020) did not affect the ruminal pH. In contrast to our findings, under in vitro conditions, pH values increased in the diets supplemented with plant oils rich in USFA compared to the control (Jalc et al., 2002). However, another study showed that addition of 3.5 % (wt wt−1) oleic acid to a diet containing 80 % lucerne and 20 % barley decreased the in vitro ruminal fluid pH value (Jalc et al., 2007).
In the current experiment, the diet containing 60 % concentrate and sunflower oil produced the lowest concentration of NH3–N and resulted in the lowest number of total protozoa. There is a positive relationship between rumen defaunation and ammonia nitrogen concentration (Machmüller et al., 1998). The low NH3–N concentration in the diet containing 60 % concentrate and sunflower oil may be associated with reduction of proteolytic protozoa that engulf the rumen bacteria and release NH3–N into the ruminal fluid (Doreau and Ferlay, 1995).
In line with the current results, under in vitro conditions, oleic acid inhibited the ruminal microbes and limited the deamination of proteins and peptides and subsequently decreased NH3–N concentration (12 h after incubation) compared with the control treatment (Wu et al., 2016). It is noteworthy that sunflower oil is a rich source of oleic acid, which might explain the lowest NH3–N concentration in the HCSO (high (60 %) concentrate diet containing sunflower oil) diet in our study. The decrease in ammonia nitrogen concentration may also be associated with an accumulation of amino acid or peptide N, which indicates that sunflower oil inhibits deamination to a greater extent than proteolysis (Wallace et al., 1981; Whetstone et al., 1981; Russell and Martin, 1984). Under in vitro conditions, decreased ammonia nitrogen concentration is accompanied by decreases in protein degradation and microbial protein synthesis (Russell and Martin, 1984; Roy et al., 2017). The decreased concentration of rumen NH3–N and the reduced protozoa population are also consistent with in vivo experiments (Eugène et al., 2004). The decreasing effect of dietary oils on ammonia production is environmentally beneficial as it decreases nitrogen excretion from ruminants (Vargas et al., 2020). There are, however, conflicting reports regarding the effect of dietary oil on ammonia production; for example, in batch culture systems, 300 mg L−1 garlic oil increased ammonia concentration (Busquet et al., 2005).
It has also been reported that when in vitro barley-based diets were supplemented with pure individual fatty acids, linoleic acid effectively reduced the protozoal number (Hristov et al., 2004). However, Váradyová et al. (2007) found that supplementing sheep diets with 5 % sunflower oil (including 53 % of C18:2) decreased only some protozoal species (Isotricha spp. and Eremoplastron dilobum), with no significant effect on the total protozoa population. A high degree of FA unsaturation inserts greater inhibitory effects on protozoa (Roy et al., 2017). When protozoa become covered by oil, their metabolic activity is inhibited, and many protozoa will die once the content of the ruminal fat is too high (Tamminga and Doreau, 1991). Decreased microbial activity due to membrane disruption can occur with unsaturated fatty acids because the double bonds alter the shape of the membrane lipid, causing these links to disrupt the membrane and negatively impacting the cell functionality (Maia et al., 2007).
In contrast to our experiment, the addition of 6 % olive, sunflower or linseed oil to high concentrate diets did not significantly affect the number of ciliate protozoa (Vargas et al., 2020). Pomegranate oil increased the number of protozoa under in vitro conditions (Maleki et al., 2016). Pomegranate oil is a rich source of punicic acid, which is an isomer of linolenic acid. Other researchers have shown that flaxseed oil, which is rich in linolenic acid, did not affect the protozoa population in cows (Doreau et al., 2009; Benchar et al., 2014).
The influence of supplemental oil on protozoa depends on the oil concentration, type, and fatty acid composition, as well as the nutrient composition of the diet (Ueda et al., 2003; Benchar et al., 2014). Inconsistencies observed between in vitro studies could be due to the incubation systems (batch vs. continuous culture) or length of incubation period (24 h in batch fermentations and 9 d in continuous culture) (Vergas et al., 2017).
In the current study, the ME content and SCFA production, estimated from the gas production data after 24 h of incubation, were not different among the treatment groups. The pH is an important factor affecting fermentation and volatile fatty acid (VFA) concentration. Hence, the lack of significant effect of the concentrate level on pH may be the reason for no difference between SCFA estimation in the current study. Furthermore, propionate-producing gram-negative bacteria are not significantly inhibited by the fatty acids (O'Brien et al., 2014), which could explain the lack of effect of oils on the estimated SCFA concentration in the present study.
In a similar study, Ishlak et al. (2014) reported that supplementation with soybean, pomegranate or blackberry oil in a continuous culture system had no influence on total VFA (Ishlak et al., 2014). In line with the present study, supplementation of the diets with 6 % olive, sunflower or linseed oil to high concentrate diets had no significant effect on total VFA production, but significant effects of oil supplementation on the production of specific VFA were noticed (Vargas et al., 2020).
At 2 % inclusion rate in the diets containing 40 % or 60 % concentrates, pomegranate and garlic oil did not adversely impact on the in vitro fermentation kinetics. No substantial differences in in vitro fermentation patterns were observed among pomegranate, garlic, and sunflower oil differing in polyunsaturated fatty acid composition.
Data will be made available upon reasonable request.
SK developed the investigation and conceptualization and did the formal analysis. GT developed the investigation and administrated the project, did the formal analysis, and wrote the original draft. AA wrote the original draft. MJZ revised the original draft.
The contact author has declared that none of the authors has any competing interests.
This study was performed according to the Iranian Council of Animal Care (1995).
Publisher's note: Copernicus Publications remains neutral with regard to jurisdictional claims in published maps and institutional affiliations.
This paper was edited by Manfred Mielenz and reviewed by Najmeh Eslamian Farsouni and one anonymous referee.
Abu Ghazaleh, A. A. and Ishlak, A.: Effects of incremental amounts of fish oil on trans fatty acids and Butyrivibrio bacteria in continuous culture fermenters, J. Anim. Physiol. Anim. Nutr., 98, 271–278, https://doi.org/10.1111/jpn.12077, 2014
Benchar, C., McAllister, T. A., Petit, H. V., and Chouinard, P. Y.: Whole flax seed and flax oil supplementation of dairy cows fed high-forage or high-concentrate diets: Effects on digestion, ruminal fermentation characteristics, protozoal populations and milk fatty acid profile, Anim. Feed Sci. Technol., 198, 117–129, https://doi.org/10.1016/j.anifeedsci.2014.10.003, 2014.
Blümmel, M. and Orskov, E. R.: Comparison of in vitro gas production and nylon bag degradability of roughages in predicting feed intake in cattle, Anim. Feed Sci. Technol., 40, 109–119, https://doi.org/10.1016/0377-8401(93)90150-I, 1993.
Broderik, G. A. and Kang, G. H.: Automated simultaneous determination of ammonia and total amino acids in ruminal fluid and in vitro media, J. Dairy Sci., 63, 64–75, https://doi.org/10.3168/jds.S0022-0302(80)82888-8, 1980.
Busquet, M., Calsamiglia, S., Ferret, A., Carro, M. D., and Kamel, C.: Effect of garlic oil and four of its compounds on rumen microbial fermentation, J. Dairy Sci., 88, 4393–4404, https://doi.org/10.3168/jds.S0022-0302(05)73126-X, 2005.
Chen, H., Wang, C., Huasai, S., and Chen, A.: Effects of dietary forage to concentrate ratio on nutrient digestibility, ruminal fermentation and rumen bacterial composition in Angus cows, Sci. Rep., 11, 17023, https://doi.org/10.1038/s41598-021-96580-5, 2021.
Dadashi, S., Mousazadeh, M., Emam-Djomeh, Z., and Mousavi, M.: Pomegranate (Punica granatum L.) seed: A comparative study on biochemical composition and oil physicochemical characteristics biochemical composition of pomegranate seed oil, Int. J. Adv. Biol., 1, 351–363, 2013.
Dehority, B. A.: Rumen Microbiology, Nottingham University Press, UK, ISBN 1-897676-99-9, 2003.
Doreau, M., Aurousseau, E., and Martin, C.: Effects of linseed lipids fed as rolled seeds, extruded seeds or oil on organic matter and crude protein digestion in cows, Anim. Feed Sci. Technol., 150, 187–196, https://doi.org/10.1016/j.anifeedsci.2008.09.004, 2009.
Doreau, M. and Ferlay, A.: Effect of dietary lipids on nitrogen metabolism in the rumen: a review, Livest. Prod. Sci., 43, 97–110, https://doi.org/10.1016/0301-6226(95)00041-I, 1995.
El-Sherbiny, M., Cieslak, A., Pers-Kamczyc, E., Szczechowiak, J., Kowalczyk, D., and Szumacher-Strabel, M.: A nanoemulsified form of oil blends positively affects the fatty acid proportion in ruminal batch cultures, J. Dairy Sci., 99, 399–407, https://doi.org/10.3168/jds.2015-9328, 2016.
Eugène, M., Archimède, H., and Sauvant, D.: Quantitative meta-analysis on the effects of defaunation of the rumen on growth, intake and digestion in ruminants, Livest. Prod. Sci., 85, 81–97, https://doi.org/10.1016/S0301-6226(03)00117-9, 2004.
Fadavi, A., Barzegar, M., and Azizi, M. H.: Determination of fatty acids and total lipid content in oilseed of 25 pomegranates varieties grown in Iran, J. Food Compos. Anal., 19, 676–680, https://https://doi.org/10.1016/j.jfca.2004.09.002, 2006.
Griinari, J. M., Corl, B. A., Lacy, S. H., Chouinard, P. Y., Nurmela, K. V., and Bauman, D. E.: Conjugated linoleic acid is synthesized endogenously in lactating dairy cows by delta(9)-desaturase, J. Nutr., 130, 2285–2291, http://https://doi.org/10.1093/jn/130.9.2285, 2000.
Hristov, A. N., Ivan, M., and McAllister, T. A.: In vitro effects of individual fatty acids on protozoal numbers and on fermentation products in ruminal fluid from cattle fed a high-concentrate, barley-based diet, J. Anim. Sci., 82, 2693–2704, https://doi.org/10.2527/2004.8292693x, 2004.
Ishlak, A., AbuGhazaleh, A. A., and Günal, M.: Short communication: Effect of blackberry and pomegranate oils on vaccenic acid formation in a single-flow continuous culture fermentation system, J. Dairy Sci., 97, 1067–1071, https://doi.org/10.3168/jds.2013-6860, 2014.
Ivan, M., Mir, P., Koenig, K., Rode, L., Neill, L., Entz, T., and Mir, Z.: Effects of dietary sunflower seed oil on rumen protozoa population and tissue concentration of conjugated linoleic acid in sheep, Small Rumin. Re., 41, 215–227, https://doi.org/10.1016/S0921-4488(01)00220-6, 2001.
Jalc, D., Kisidayova, S., and Nerud, F.: Effect of plant oils and organic acids on rumen fermentation in vitro, Folia Microbiol., 47, 171–177, https://doi.org/10.1007/BF02817677, 2002.
Jalc, D., Certik, M., Kundrikova, K., and Namestkova, P.: Effect of unsaturated C-18 fatty acids (oleic, linoleic and alpha-linolenic acid) on ruminal fermentation and production of fatty acid isomers in an artificial rumen, Vet Med., 52, 87–94, https://doi.org/10.17221/2012-VETMED, 2007.
Jenkins, T. C.: Lipid metabolism in the rumen, J. Dairy Sci., 76, 3851–3863, https://doi.org/10.3168/jds.S0022-0302(93)77727-9, 1993.
Khoddami, A. and Roberts, T.: Pomegranate oil as a valuable pharmaceutical and nutraceutical, Lipid Technol., 27, 40–42, https://doi.org/10.1002/lite.201500002, 2015.
Machmüller, A., Ossowski, D., Wanner, M., and Kreuzer, M.: Potential of various fatty feeds to reduce methane release from rumen fermentation in vitro (Rusitec), Anim. Feed Sci. Technol., 71, 117–130, https://doi.org/10.1016/S0377-8401(97)00126-0, 1998.
Maia, M. R. G., Chaudhary, L. C., Figueres, L., and Wallace, R. J.: Metabolism of polyunsaturated fatty acids and their toxicity to the microflora of the rumen, Antonie Van Leeuwenhoek, 91, 303–314, https://doi.org/10.1007/s10482-006-9118-2, 2007.
Makkar, H. P. S.: In vitro screening of feed resources for efficiency of microbial protein synthesis, in: In vitro screening of plant resources for extra nutritional attributes in ruminants, edited by: Vercoe, P. E., Makkar, H. P. S., and Schlink, A. C., 112 pp., IAEA, Dordrecht, the Netherlands, https://doi.org/10.1007/978-90-481-3297-3, 2010.
Maleki, E., Meng, G. Y., Faseleh Jahromi, M., Jorfi, R., Khoddami, A, and Ebrahimi, M.: Pomegranate seed oil rich in conjugated linolenic acids reduces in vitro methane production, S. Afr. J. Anim. Sci., 46, 327–335, https://doi.org/10.4314/sajas.v46i3.13, 2016.
McDonald, I. A.: Revised model for the estimation of protein degradability in the rumen, J. Agric. Sci., 96, 251–252, https://doi.org/10.1017/S0021859600032081, 1981.
McDougall, E. I.: Studies on ruminant saliva, I. The composition and output of sheep's saliva, Biochem. J., 43, 99–109, 1948.
Menke, K. H. and Steingass, H.: Estimation of energetic feed value obtained from chemical analysis and in vitro production using rumen fluid, J. Anim. Res., 28, 7–55, 1988.
Muetzel, S., Hunt, C., and Tavendale, M. H.: A fully automated incubation system for the measurement of gas production and gas composition, Anim. Feed Sci. Technol., 196, 1–11, https://doi.org/10.1016/j.anifeedsci.2014.05.016, 2014.
Nagaraja, T. G., Newbold, C. J., van Nevel, C. J., and Demeyer, D. I.: Manipulation of ruminal fermentation in The rumen microbial ecosystem, edited by: Hobson, P. N., Stewart CS, https://doi.org/10.1007/978-94-009-1453-7_13, 1997.
NRC: Nutrient Requirements of Dairy Cattle, 7th Edn., National Academy Press, Washington, DC, USA, ISBN 0-309-06997-1, 2001.
O'Brien, A. M., Navarro-Villa, A., Purcell, P.J., Boland, T. M., and O'Kiely, A. P.: Reducing in vitro rumen methanogenesis for two contrasting diets using a series of inclusion rates of different additives, Anim. Prod. Sci., 54, 41–157, https://doi.org/10.1071/AN12204, 2014.
Palmquist, D. L. and Jenkins, T. C.: Fat in lactation rations: Review, J. Dairy Sci., 63, 1–14, https://doi.org/10.3168/jds.S0022-0302(80)82881-5, 1980.
Patra, A. K.: The effect of dietary fats on methane emissions, and its other effects on digestibility, rumen fermentation and lactation performance in cattle: A meta-analysis, Livest. Sci., 155, 244–254, https://doi.org/10.1016/j.livsci.2013.05.023, 2013.
Roy, A., Mandal, G. P., and Patra, A. K.: Effects of different vegetable oils on rumen fermentation and conjugated linoleic acid concentration in vitro, Vet. World, 10, 11–16, https://doi.org/10.14202/vetworld.2017.11-16, 2017.
Russell, J. B. and Martin, S. A.: Effects of various methane inhibitors on the fermentation of amino acids by mixed rumen microorganisms in vitro, J. Anim. Sci., 59, 1329–1338, https://doi.org/10.2527/jas1984.5951329x, 1984.
Sethi, N., Kaura, S., Dilbaghi, N., Parle, M., and Pal, M.: Garlic: a pungent wonder from nature, Int. Res. J. Pharm., 5, 523–529, 2014.
Saliba, L., Gervais, R., Lebeuf, Y., and Chouinard, P. Y.: Effect of feeding linseed oil in diets differing in forage to concentrate ratio: 1. Production performance and milk fat content of biohydrogenation intermediates of α-linolenic acid, J. Dairy Res., 81, 82–90, https://doi.org/10.1017/S0022029913000691, 2014.
Tamminga, S. and Doreau, M.: Lipids and rumen digestion, in: Rumen Microbial Metabolism and Ruminal Digestion, edited by: Jouany, J. P., INRA, Paris, France, ISBN: 2738003451, 9782738003454, 1991.
Theodorou, M. K., Williams, B. A., Dhanoa, M. S., McAllen, A. B., and France, J. A.: Simple gas production method using a pressure transducer to determine the fermentation kinetics of ruminant feeds, Anim. Feed Sci. Technol., 48, 185–197, https://doi.org/10.1016/0377-8401(94)90171-6, 1994.
Tsiaganis, M. C., Laskari, K., and Melissari, E.: Fatty acid composition of Allium species lipids, J. Food Compos. Anal., 19, 620–627, https://doi.org/10.1016/j.jfca.2005.06.003, 2006.
Ueda, K., Ferlay, A., Chabrot, J., Loor, J. J. Chilliard, Y., and Doreau, M.: Effect of linseed oil supplementation on ruminal digestion in dairy cows fed diets with different forage: concentrate ratios, J. Dairy Sci., 86, 3999–4007, https://doi.org/10.3168/jds.S0022-0302(03)74011-9, 2003.
Váradyová, Z., Kišidayová, S., Siroka, P., and Jalč, D.: Fatty acid profiles of rumen fluid from sheep fed diets supplemented with various oils and effect on the rumen ciliate population, Czech J. Anim Sci., 52, 399–406, https://doi.org/10.17221/2322-CJAS, 2007.
Vargas, J. E., Andrés, S., López-Ferreras, L., Snelling, T. J., Yáñez-Ruíz, D. R., García-Estrada, C., and López, S.: Effect of sunflower and marine oils on ruminal microbiota, in vitro fermentation and digesta fatty acid profile, Front. Microbiol., 8, 1–15, https://doi.org/10.3389/fmicb.2017.01124, 2017.
Vargas, J. E., Andrés, S., López-Ferreras, L., Snelling, T. J., Yáñez-Ruíz, D. R., García-Estrada, C., and López, S.: Dietary supplemental plant oils reduce methanogenesis from anaerobic microbial fermentation in the rumen, Sci. Rep., 10, 1613, https://doi.org/10.1038/s41598-020-58401-z, 2020.
Wallace, R. J., Czerkawski, J. W., and Breckenridge, G.: Effect of monensin on the fermentation of basal ration in the Rumen Simulation Technique (RUSITEC), Br. J. Nutr., 46, 131–148, https://doi.org/10.1079/BJN19810016, 1981.
Whetstone, H. D., Davis, C. L., and Bryant, M. P.: Effect of monensin on breakdown of protein by ruminal microorganisms in vitro, J. Anim. Sci., 55, 803–809, https://doi.org/10.2527/jas1981.533803x, 1981.
Wu, D., Xu, L., Tang, S., Guan, L., He, Z., Guan, Y., Tan, Z., Han, X., Zhou, C., Kang, J., and Wang, M.: Influence of oleic acid on rumen fermentation and fatty acid formation in vitro, Plos ONE, 11, 1–13, https://doi.org/10.1371/journal.pone.0156835, 2016.
Zhang, C. M., Guo, Y. Q., Yuan, Z. P., Wu, Y. M., Wamg, J. K., Liu, J. X., and Zhu, W. Y.: Effect of octadeca carbon fatty acids on microbial fermentation, methanogenesis and microbial flora in vitro, Anim. Feed Sci. Technol., 146, 259–269, 2008.