the Creative Commons Attribution 4.0 License.
the Creative Commons Attribution 4.0 License.
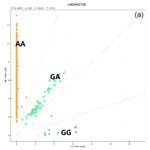
Polymorphisms of the HRG, FETUB, and GUCY1A1 genes and their association with litter size in sheep
Zizhen Ren
Xiaoyun He
Xiangyu Wang
Mingxing Chu
Litter size is one of the key factors affecting the efficiency of sheep breeding, and previous studies found that the HRG, FETUB, and GUCY1A1 genes were closely related to litter size in sheep. This experiment aims to explore the polymorphisms of the g.405442728A>G locus of the HRG gene, the g.421655951C>T locus of the FETUB gene, and the g.414050897G>C locus of the GUCY1A1 gene and their association with sheep litter size. The MassARRAY® single-nucleotide polymorphism (SNP) genotyping technique was used to detect the polymorphisms of these loci in five sheep breeds, i.e., Small-tailed Han sheep, Hu sheep, Cele black sheep, Sunite sheep, and Bamei mutton sheep. In addition, the association between the polymorphisms of these genes and the litter size of Small-tailed Han sheep was also analyzed. The results showed that the g.405442728A>G locus of the HRG gene was moderately polymorphic (0.25 < PIC <0.5) in both monotocous and polytocous sheep breeds; the g.421655951C>T locus of the FETUB gene was lowly polymorphic (PIC <0.25) in five sheep breeds; the g.414050897G>C locus of GUCY1A1 showed moderately polymorphism in Small-tailed Han sheep (0.25 ≤ PIC <0.5) and low polymorphism in four other sheep breeds (PIC <0.25). The chi-squared test results showed that the g.405442728A>G locus of the HRG gene was in the Hardy–Weinberg equilibrium state in five sheep breeds (P>0.05). The g.421655951 C>T locus of the FETUB gene and the g.414050897G>C locus of the GUCY1A1 gene were in the Hardy–Weinberg equilibrium state in Small-tailed Han sheep (P>0.05) and in the Hardy–Weinberg disequilibrium state in other sheep breeds (P<0.05). The association analysis showed that the g.405442728A>G locus of the HRG gene and the g.421655951C>T locus of the FETUB gene had a significant impact on the litter size of sheep (P<0.05), while the g.414050897G>C locus of the GUCY1A1 gene had no significant impact on the litter size (P>0.05). In summary, the HRG gene and the FETUB gene can be used as potential molecular markers for the selection of the litter size in sheep.
- Article
(1267 KB) - Full-text XML
- BibTeX
- EndNote
Fecundity is one of the most important economic traits of sheep. Sheep with high fecundity showed 2 to 3 times the lamb production efficiency and economic benefits compared with sheep with low fecundity. The premise of improving fertility is successful fertilization, but many factors can lead to fertilization failure. For example, zona pellucida sclerosis occurs during the spontaneous maturation of mouse oocytes (Felici et al., 1985), and sperm penetration can be affected by its sclerosis, which will prevent fertilization (Schroeder et al., 1990). A complete ovary is also a prerequisite for improving fertility, and blood vessels are closely related to the integrity of the ovary (McFee and Cupp, 2013). Few sheep show the characteristics of high fecundity and perennial estrus around the world, and the researchers found the main genes affecting fecundity in a small number of high-fecundity sheep breeds. It has been proven that the formation and changes in endometrial blood vessels may lead to early-pregnancy abortion (Lash et al., 2012; Banerjee et al., 2013). However, there are far more than a few genes that affect fecundity, and new genes need to be discovered constantly.
Histidine-rich glycoprotein (HRG) is composed of 75 kDa glycoprotein, which is synthesized in the liver and can be circulated in the plasma. It was firstly isolated from human serum in 1972 (Haupt and Heimburger, 1972) and then found in the plasma of rats, rabbits, chicken, cattle, and other vertebrates. HRG is usually classified as a member of the cysteine protease inhibitor (CPI), although some researchers suggested that HRG should be classified as a new family in the cystatin superfamily (Koide and Odani, 1987). The exact biological molecular function of HRG is still unclear, but HRG has a prominent histidine-rich domain, which may be the basis of the interaction between HRG and many molecules, affecting neutrophils, red blood cells, and vascular endothelial cells (Nishibori, 2022). Some research results show that the C633T polymorphism of the HRG gene may have an impact on ovarian reactivity, oocyte quality, and the development of fertilized eggs in Chinese women (Jin et al., 2015) and play an important role in recurrent abortion (Lindgren et al., 2013). A single-nucleotide polymorphism (SNP) (A1042G) of the HRG gene is also related to recurrent abortion (Elenis et al., 2014). HRG plays an important role in angiogenesis, immune function, and coagulation processes, which are inextricably linked to pregnancy (Elenis et al., 2014). In addition, the HRG gene has at least 10 SNPs (UniProt Consortium, 2014), among which C633T may have an impact on the fecundity of sheep.
FETUB is a novel liver cytokine that belongs to the cystatin superfamily, which, like the HRG gene, belongs to the cystatin cysteine protease inhibitor superfamily (Jung et al., 2015; Meex et al., 2015). The potential functions of FETUB have not been clearly explained, but it has some similarities to fetuin. FETUB can play a role before fertilization, making the zona pellucida harden only early (Dietzel et al., 2013; Stoecker et al., 2014), maintaining the permeability of the zona pellucida, and playing an important role in the fertilization process. After fertilization, a series of changes will cause the zona pellucida to harden (Burkart et al., 2012). Reversible infertility of female mice can be achieved by regulating FETUB (Floehr et al., 2017). Some studies have shown that FETUB has a certain expression in rodents and primates during the second trimester of pregnancy (Olivier et al., 2000). Sperm proteins are very important in oocyte maturation, fertilization, and early embryonic development (Binsila et al., 2021). GUCY1A1 is a gene encoding soluble guanylate cyclase (sGC). In goats, sheep (Zhu et al., 2020), pigs (Roca et al., 2020), and other animals, GUCY1A1 affects coronary artery disease (Kessler et al., 2017) and hypertension (Curtis, 2021), but these influences may change with other factors such as people's age (Malinowski et al., 2022a). GUCY1A1 also affects platelet adhesion and thrombosis (Malinowski et al., 2022a).
To further understand the effects of the above three genes on sheep fecundity, Small-tailed Han sheep, Hu sheep, Cele black sheep, Sunite sheep, and Bamei mutton sheep were selected for this study. We screened SNPs in the above five sheep breeds and genotyped them using the methylation mass spectrometry® platform. The distribution of each genotype in each sheep population was obtained, and the SNP loci related to reproductive traits were expected to provide valuable genetic markers for sheep genetic breeding.
2.1 Animals
Five sheep breeds were used in this experiment, i.e., 384 Small-tailed Han sheep, 96 Hu sheep, 96 Cele black sheep, 96 Sunite sheep, and 96 Bamei mutton sheep (Table 1). Blood was collected from the jugular vein and anticoagulated with glucose citrate at −20°. At the same time, the litter size of Small-tailed Han sheep was recorded.
2.2 Genotyping
Genotyping of the g.405442728 A>G locus of HRG, the g.421655951 C>T locus of FETUB, and the g.414050897 G>C locus of GUCY1A1 in polytocous and monotocous sheep breeds was identified by the MassARRAY® SNP genotyping technique.
2.3 Data analysis
Microsoft Excel 2021 software was used to count the genotype frequency, gene frequency, polymorphism information content (PIC), heterozygosity (He), and effective allele number (Ne). The Hardy–Weinberg equilibrium test was also conducted. A linear model, , was applied to analyze the association of genotypes with litter size as in our previous study (He et al., 2019). Prediction of protein secondary structure was conducted by online tools: http://rna.tbi.univie.ac.at//cgi-bin/RNAWebSuite/RNAfold.cgi (last access: 18 September 2023).
3.1 Analysis of HRG, FETUB, and GUCY1A1 polymorphism
The results showed that the HRG, FETUB, and GUCY1A1 genes all have three genotypes in Small-tailed Han sheep (Fig. 1).
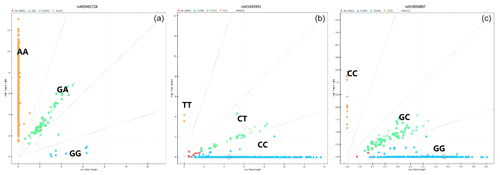
Figure 1The genotyping results of the HRG, FETUB, and GUCY1A1 genes. (Note: a – the g.405442728A>G locus representing the HRG gene; b – the g.421655951C>T locus representing the FETUB gene; c – the g.414050897G>A locus representing the GUCY1A1 gene).
The genotypic frequencies of the g.405442728 A>G locus of the HRG gene and the g.421655951 C>T locus of the FETUB gene were significantly different between polytocous and monotocous sheep breeds (P<0.01), and there were no significant differences in allele frequencies (P>0.05). The dominant alleles in sheep breeds were A and C, respectively. The genotype frequency and allele frequency of the g.414050897 G>C locus of the GUCY1A1 gene were significantly different between polytocous and monotocous sheep breeds (P<0.01), and the dominant allele in polytocous and monotocous sheep breeds was G (Fig. 2).
Table 2Genotypic and allelic frequencies of HRG, FETUB, and GUCY1A1 polymorphism in polytocous and monotocous sheep breeds.
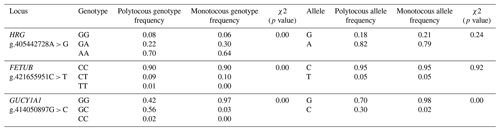
It can be seen from Table 3 that the g.405442728A>G locus of the HRG gene showed moderate polymorphism in Small-tailed Han sheep, Hu sheep, Cele black sheep, Sunite sheep, and Bamei mutton sheep (0.25< PIC <0.5). The g.421655951C>T locus of the FETUB gene showed low polymorphism in five sheep breeds (PIC <0.25). The g.414050897G>C locus of GUCY1A1 showed moderate polymorphism in Small-tailed Han sheep (0.25< PIC <0.5) and low polymorphism in Hu sheep, Cele black sheep, Sunite sheep, and Bamei mutton sheep (PIC <0.25). The chi-squared test results showed that the g.405442728A>G locus of the HRG gene was in the Hardy–Weinberg equilibrium state in five sheep breeds (P>0.05). The g.421655951C>T locus of the FETUB gene and the g.414050897G>C locus of the GUCY1A1 gene were in the Hardy–Weinberg equilibrium state in Small-tailed Han sheep (P>0.05) and in the Hardy–Weinberg disequilibrium state in other sheep breeds (P<0.05).
Table 3Population genetic analyses of the HRG, FETUB, and GUCY1A1 polymorphisms in different sheep breeds.
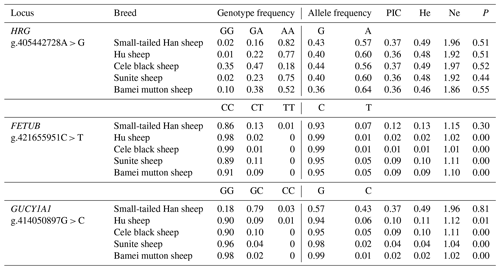
Note: monotocous: Sunite and Bamei mutton sheep; polytocous: Small-tailed Han sheep, Hu sheep, and Cele black sheep; PIC: polymorphic information content; He: heterozygosity; Ne: number of effective alleles.
3.2 Association analysis of HRG, FETUB, and GUCY1A1 polymorphism and litter size of Small-tailed Han sheep
The association analysis between the genotypes of the three polymorphic loci of the HRG, FETUB, and GUCY1A1 genes and the litter size of three parities of Small-tailed Han sheep was carried out. There was a significant correlation between the polymorphism of the g.405442728A>G locus of HRG and the litter size of the first and third parities of Small-tailed Han sheep (P<0.05). The litter size of GG-type sheep in the first parity was significantly higher than that of the GA and AA types, and the litter size of GG-type sheep in the third parity was significantly lower than that of the AA type; there was a significant correlation between the polymorphism of the g.421655951C>T locus of FETUB and the litter size of the third parity of Small-tailed Han sheep (P<0.01). In the third parity, the litter size of CT-type sheep was significantly lower than that of CC-type sheep. The mutation of the GUCY1A1 gene had no significant influence on the litter size (P>0.05) (Table 4).
3.3 Amino acid predictions of the HRG, FETUB, and GUCY1A1 genes
To further study the effect of mutation on the structure of genes, the sequence of HRG, FETUB, and GUCY1A1 before and after the mutation was used for amino acid prediction (Fig. 2). The minimum free energy of HRG, FETUB and GUCY1A1 before and after mutation was −416.30, −416.20, −338.70, −335.90, −603.80, and −602.90 kcal mol−1, respectively. The free energy of a spontaneous process will be reduced, so the transformation process of the above three genes can be carried out spontaneously.
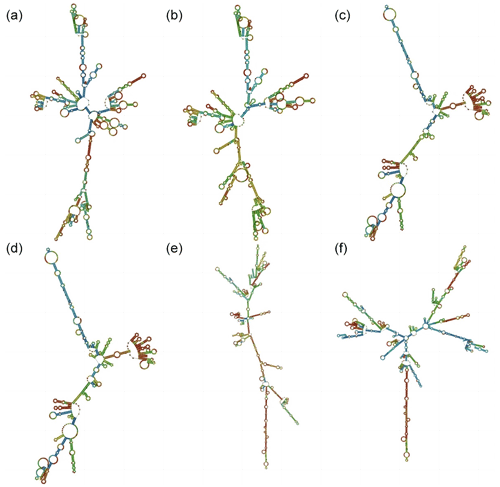
Figure 2Secondary structures before and after gene mutation. (a)–(b) The secondary structure of HRG before and after mutation. (c)–(d) The secondary structure of FETUB before and after mutation. (e)–(f) The secondary structure of GUCY1A1 before and after mutation. The different colors represent different base-pairing probabilities, where red > green > blue.
Any mutation of the gene locus is closely related to the role of the gene in the body. This article only involves one aspect for subsequent reference.
HRG seems to be an adaptive-molecule, histidine-rich glycoprotein (HRG) in plasma that can inhibit S100A8/A9-mediated melanoma cell organ metastasis, and plasma protein HRG plays a significant role in protecting the brain and lungs from melanoma metastasis (Tomonobu et al., 2022). HRG is also involved in tumor growth regulation (Karrlander et al., 2009; Tugues et al., 2012; Pan et al., 2022). HRG is a multi-domain molecule that interacts with a variety of gametes. The multi-domain structure of HRG indicates that the molecule can act as an adaptor protein to aggregate different ligands under certain conditions to play different roles (Jones et al., 2005). A preliminary study found that HRG is closely related to the maintenance and establishment of pregnancy (Nordqvist et al., 2011), and the gene exists in the structure of the female reproductive tract (Nordqvist et al., 2010). The existing results show that HRG is an important mammary gland mitogen for lactation (Li et al., 2002) and plays an important role in reproduction. Follicular fluid is rich in histidine glycoprotein. If its concentration in follicular fluid is lower than 35.80 mg dL−1, the possibility of obtaining live birth is greatly reduced (Zhang et al., 2021). Tsuchida Straten et al. (2005) reported that mice with HRG deletion can survive and reproduce without obvious abnormalities, but in some cases HRG deletion will have a greater impact (Wakabayashi, 2013). Our findings showed that the g.405442728A>G locus of the HRG gene had a significant impact on the litter size of Small-tailed Han sheep (P<0.05). This indicates that the HRG gene can be used as a potential molecular marker for the litter size of Small-tailed Han sheep, which is consistent with the previous conclusion that the HRG gene is closely related to reproduction (Nordqvist et al., 2011; Lindgren et al., 2013).
Fetuin-B is a newly discovered member of the cystatin cysteine protease inhibitor superfamily (Jung et al., 2015; Meex et al., 2015). Studies have found that FETUB is related not only to coronary artery disease (Zhu et al., 2017) but also to reproduction, zona pellucida sclerosis, and sperm protein, thus affecting pregnancy and fertility. Reproduction is a complex process that is affected by many processes. Metabolic stability is a very important factor affecting sheep reproduction, and FETUB is an energy metabolism gene (Kralisch et al., 2017). Some studies have shown that the level of FETUB significantly increased during pregnancy (Simjak et al., 2018) (which may be related to the mechanism of pregnancy protection), endometrial translocation will lead to decreased fertility, and FETUB is related to this process (Cao et al., 2022). One study in mice found that FETUB is important for fertilization but is not so important for late pregnancy (Floehr et al., 2016). Stocker et al. (2014) found that FETUB maintains mammalian gamete fusion by inhibiting aspergillin, which is the switch triggering zona pellucida hardening. FETUB also plays a role in human reproduction, the FETUB content of women is higher than that of men, and estrogen or progesterone may be related to its regulation (Denecke et al., 2003). Studies have shown that the level of FETUB in plasma seriously affects the fertilization rate (Zhang et al., 2022). Our findings showed that the g.421655951C>T locus of the FETUB gene had a very significant impact on the litter size of Small-tailed Han sheep (P<0.01). This result is consistent with the previous results, which proves that the FETUB gene plays an important role in animal reproduction.
Guanylate cyclase 1 soluble subunit α1 (GUCY1A1) can affect vascular reactivity and tubular function, thereby affecting thrombosis and leading to coronary artery disease (Malinowski et al., 2022). The SNP marker of GUCY1A1 can also be used for the reproduction of Lufan black sheep (Liu et al., 2019). Studies have confirmed that the g.43266624C>T locus of the GUCY1A1 gene is significantly related to the litter size in the field sheep (Ma et al., 2019). This study found that the g.414050897G>C locus of the GUCY1A1 gene had no significant impact on the litter size of Small-tailed Han sheep (P<0.05). The results of this experiment showed that there was no significant correlation between this locus and sheep reproduction, and the specific relationship needs to be further verified, which also provides a reference for further study of the role of the GUCY1A1 gene.
In this study, the g.405442728A>G locus of the HRG gene exhibited moderate polymorphism (0.25< PIC <0.5) in both monotocous and polytocous sheep breeds, indicating that this locus has great selection potential in the five populations. Due to the existence of genetic variation, the above conclusion may not be desirable. However, among all the breeds tested, only Small-tailed Han sheep had a significant relationship between litter size and genotype. Therefore, it is accurate to say that the HRG gene can be used as a molecular marker to increase litter size in Small-tailed Han sheep. In Small-tailed Han sheep, the g.405442728A>G locus of the HRG gene and the g.421655951C>T locus of the FETUB gene had a significant effect on the litter size (P<0.05), while the g.414050897G>C locus of the GUCY1A1 gene had no significant effect (P>0.05). Therefore, we can use the HRG and FETUB genes as candidate genes for the selection of litter size in sheep breeding.
No data sets were used in this article.
XH and ZR performed the experiments, analyzed the data, and wrote the first draft. XW contributed to the tissue, serum collection, and pretreatment of the samples. MC contributed to the experimental design and manuscript revision.
The contact author has declared that none of the authors has any competing interests.
All the experimental procedures mentioned in the present study were approved by the Science Research Department (in charge of animal welfare issues) of the Institute of Animal Science (IAS-CAAS) (Beijing, China). Ethical approval was given by the Animal Ethics Committee of the IAS (IAS2021-24).
Publisher’s note: Copernicus Publications remains neutral with regard to jurisdictional claims made in the text, published maps, institutional affiliations, or any other geographical representation in this paper. While Copernicus Publications makes every effort to include appropriate place names, the final responsibility lies with the authors.
This research was funded by the National Natural Science Foundation of China (grant no. 32172704), the Agricultural Science and Technology Innovation Program of China (CAAS-ZDRW202106 and ASTIP-IAS13), and the China Agriculture Research System of MOF and MARA (CARS-38).
This research has been supported by the National Natural Science Foundation of China (grant no. 32172704).
This paper was edited by Joachim Weitzel and reviewed by two anonymous referees.
Banerjee, P., Jana, S. K., Pasricha, P., Ghosh, S., Chakravarty, B., and Chaudhury, K.: Proinflammatory cytokines induced altered expression of cyclooxygenase-2 gene results in unreceptive endometrium in women with idiopathic recurrent spontaneous miscarriage, Fertil. Steril., 99, 179–187, https://doi.org/10.1016/j.fertnstert.2012.08.034, 2013.
Binsila, B. K., Archana, S. S., Ramya, L., Swathi, D., Selvaraju, S., Gowda, N. K. S., Pal, D. T., Rafay, A., and Bhatta, R.: Elucidating the processes and pathways enriched in buffalo sperm proteome in regulating semen quality, Cell Tissue Res., 383, 881–903, https://doi.org/10.1007/s00441-020-03303-9, 2021.
Burkart, A. D., Xiong, B., Baibakov, B., Jimenez-Movilla, M., and Dean, J.: Ovastacin, a cortical granule protease, cleaves ZP2 in the zona pellucida to prevent polyspermy, J. Cell Biol., 197, 37–44, https://doi.org/10.1083/jcb.201112094, 2012.
Cao, X. L., Song, J. Y., and Sun, Z. G.: Quantitative label-free proteomic analysis of human follicle fluid to identify novel candidate protein biomarker for endometriosis-associated infertility, J. Proteomics, 266, 104680, https://doi.org/10.1016/j.jprot.2022.104680, 2022.
Curtis, D.: Analysis of 200,000 exome-sequenced UK biobank subjects implicates genes involved in increased and decreased risk of hypertension, Pulse (Basel), 9, 17–29, https://doi.org/10.1159/000517419, 2021.
Denecke, B., Graber, S., Schafer, C., Heiss, A., Woltje, M., and Jahnen-Dechent, W.: Tissue distribution and activity testing suggest a similar but not identical function of fetuin-B and fetuin-A, Biochem. J., 376, 135–145, https://doi.org/10.1042/BJ20030676, 2003.
Dietzel, E., Wessling, J., Floehr, J., Schafer, C., Ensslen, S., Denecke, B., Rosing, B., Neulen, J., Veitinger, T., Spehr, M., Tropartz, T., Tolba, R., Renne, T., Egert, A., Schorle, H., Gottenbusch, Y., Hildebrand, A., Yiallouros, I., Stocker, W., Weiskirchen, R., and Jahnen-Dechent, W.: Fetuin-B, a liver-derived plasma protein is essential for fertilization, Dev. Cell, 25, 106–112, https://doi.org/10.1016/j.devcel.2013.03.001, 2013.
Elenis, E., Lindgren, K. E., Karypidis, H., Skalkidou, A., Hosseini, F., Bremme, K., Landgren, B. M., Skjoldebrand-Sparre, L., Stavreus-Evers, A., Sundstrom-Poromaa, I., and Akerud, H.: The histidine-rich glycoprotein A1042G polymorphism and recurrent miscarriage: a pilot study, Reprod. Biol. Endocrin., 12, 70, https://doi.org/10.1186/1477-7827-12-70, 2014.
Felici, M. D., Salustri, A., and Siracusa, G.: “Spontaneous” hardening of the zona pellucida of mouse oocytes during in vitro culture. ii. the effect of follicular fluid and glycosaminoglycans, Gamete Res., 12, 227–235, https://doi.org/10.1002/mrd.1120120302, 1985.
Floehr, J., Dietzel, E., Neulen, J., Rosing, B., Weissenborn, U., and Jahnen-Dechent, W.: Association of high fetuin-B concentrations in serum with fertilization rate in IVF: a cross-sectional pilot study, Hum. Reprod , 31, 630–637, https://doi.org/10.1093/humrep/dev340, 2016.
Floehr, J., Dietzel, E., Schmitz, C., Chappell, A., and Jahnen-Dechent, W.: Down-regulation of the liver-derived plasma protein fetuin-B mediates reversible female infertility, Mol. Hum. Reprod, 23, 34–44, https://doi.org/10.1093/molehr/gaw068, 2017.
Haupt, H. and Heimburger, N.: Human serum proteins with high affinity for carboxymethylcellulose. I. Isolation of lysozyme, C1q and 2 unknown-globulins, Hoppe-Seyler's Zeitschrift fur physiologische Chemie, 353, 1125–1132, https://doi.org/10.1515/bchm2.1972.353.2.1125, 1972.
He, X., Zhang, Z., Liu, Q., and Chu, M.: Polymorphisms of the melatonin receptor 1A gene that affects the reproductive seasonality and litter size in Small Tail Han sheep, Reprod. Domest. Anim., 54, 1400–1410, https://doi.org/10.1111/rda.13538, 2019.
Jin, B. L., Niu, Z. H., and Feng, Y.: Polymorphism of histidine-rich glycoprotein affected ovarian response in Chinese women: a pilot study, J. Reprod. Med., 24, 850–854, https://doi.org/10.3969/j.issn.1004-3845.2015.10.018, 2015.
Jones, A. L., Hulett, M. D., and Parish, C. R.: Histidine-rich glycoprotein: A novel adaptor protein in plasma that modulates the immune, vascular and coagulation systems, Immunol. Cell Biol., 83, 106–118, https://doi.org/10.1111/j.1440-1711.2005.01320.x, 2005.
Jung, S. H., Won, K., Lee, K. P., Kim, H. J., Seo, E. H., Lee, H. M., Park, E. S., Lee, S. H., and Kim, B.: The serum protein fetuin-B is involved in the development of acute myocardial infarction, Clin. Sci., 129, 27–38, https://doi.org/10.1042/CS20140462, 2015.
Karrlander, M., Lindberg, N., Olofsson, T., Kastemar, M., Olsson, A. K., and Uhrbom, L.: Histidine-rich glycoprotein can prevent development of mouse experimental glioblastoma, PLoS ONE, 4, e8536, https://doi.org/10.1371/journal.pone.0008536, 2009.
Kessler, T., Wobst, J., Wolf, B., Eckhold, J., Vilne, B., Hollstein, R., Von Ameln, S., Dang, T. A., Sager, H. B., Moritz Rumpf, P., Aherrahrou, R., Kastrati, A., Bjorkegren, J. L. M., Erdmann, J., Lusis, A. J., Civelek, M., Kaiser, F. J., and Schunkert, H.: Functional characterization of the GUCY1A3 coronary artery disease risk locus, Circulation, 136, 476–489, https://doi.org/10.1161/CIRCULATIONAHA.116.024152, 2017.
Koide, T. and Odani, S.: Histidine-rich glycoprotein is evolutionarily related to the cystatin superfamily. Presence of two cystatin domains in the N-terminal region, Febs. Lett., 216, 17–21, https://doi.org/10.1016/0014-5793(87)80748-2, 1987.
Kralisch, S., Hoffmann, A., Lossner, U., Kratzsch, J., Bluher, M., Stumvoll, M., Fasshauer, M., and Ebert, T.: Regulation of the novel adipokines/hepatokines fetuin A and fetuin B in gestational diabetes mellitus, Metab. Clin. Exp., 68, 88–94, https://doi.org/10.1016/j.metabol.2016.11.017, 2017.
Lash, G. E., Innes, B. A., Drury, J. A., Robson, S. C., Quenby, S., and Bulmer, J. N.: Localization of angiogenic growth factors and their receptors in the human endometrium throughout the menstrual cycle and in recurrent miscarriage, Hum. Reprod., 27, 183–195, https://doi.org/10.1093/humrep/der376, 2012.
Li, L., Cleary, S., Mandarano, M. A., Long, W., Birchmeier, C., and Jones, F. E.: The breast proto-oncogene, HRGalpha regulates epithelial proliferation and lobuloalveolar development in the mouse mammary gland, Oncogene, 21, 4900–4907, https://doi.org/10.1038/sj.onc.1205634, 2002.
Lindgren, K. E., Karehed, K., Karypidis, H., Hosseini, F., Bremme, K., Landgren, B. M., Skjoldebrand-Sparre, L., Stavreus-Evers, A., Sundstrom-Poromaa, I., and Akerud, H.: Histidine-rich glycoprotein gene polymorphism in patients with recurrent miscarriage, Acta Obstet. Gyn. Scan., 92, 974–977, https://doi.org/10.1111/aogs.12155, 2013.
Liu, W., Fang, C., Ma, H., Liu, L., Wang, Q., Cao, H., Lv, S., Yu, Q., and Cao, X.: New guanylate cyclase 1 soluble subunit alpha 1 (GUCY1A1) gene-specific single nucleotide polymorphism (SNP) marker, useful for breeding of black sheep of Turpan, CN109735634-A, https://webofscience.clarivate.cn/wos/alldb/full-record/DIIDW:201945152Y (last access: 1 April 2024), 2019.
Ma, H., Fang, C., Liu, L., Wang, Q., Aniwashi, J., Sulaiman, Y., Abudilaheman, K., and Liu, W.: Identification of novel genes associated with litter size of indigenous sheep population in Xinjiang, China using specific-locus amplified fragment sequencing technology, PeerJ, 7, e8079, https://doi.org/10.7717/peerj.8079, 2019.
Malinowski, D., Zawadzka, M., Safranow, K., Drozdzik, M., and Pawlik, A.: SELL and GUCY1A1 gene polymorphisms in patients with unstable angina, Biomedicines, 10, 2494, https://doi.org/10.3390/biomedicines10102494, 2022.
McFee, R. M. and Cupp, A. S.: Vascular contributions to early ovarian development: potential roles of VEGFA isoforms, Reprod. Fertil. Dev., 25, 333–342, https://doi.org/10.1071/RD12134, 2013.
Meex, R. C., Hoy, A. J., Morris, A., Brown, R. D., Lo, J. C., Burke, M., Goode, R. J., Kingwell, B. A., Kraakman, M. J., Febbraio, M. A., Greve, J. W., Rensen, S. S., Molloy, M. P., Lancaster, G. I., Bruce, C. R., and Watt, M. J.: Fetuin B is a secreted hepatocyte factor linking steatosis to impaired glucose metabolism, Cell Metab., 22, 1078–1089, https://doi.org/10.1016/j.cmet.2015.09.023, 2015.
Nishibori, M.: Novel aspects of sepsis pathophysiology: NETs, plasma glycoproteins, endotheliopathy and COVID-19, J. Pharmacol. Sci, 150, 9–20, https://doi.org/10.1016/j.jphs.2022.06.001, 2022.
Nordqvist, S., Karehed, K., Hambiliki, F., Wanggren, K., Stavreus-Evers, A., and Akerud, H.: The presence of histidine-rich glycoprotein in the female reproductive tract and in embryos, Reprod. Sci., 17, 941–947, https://doi.org/10.1177/1933719110374366, 2010.
Nordqvist, S., Karehed, K., Stavreus-Evers, A., and Akerud, H.: Histidine-rich glycoprotein polymorphism and pregnancy outcome: a pilot study, Reprod. Biomed. Online, 23, 213–219, https://doi.org/10.1016/j.rbmo.2011.04.004, 2011.
Olivier, E., Soury, E., Ruminy, P., Husson, A., Parmentier, F., Daveau, M., and Salier, J. P.: Fetuin-B, a second member of the fetuin family in mammals, Biochem. J., 350, 589–597, https://doi.org/10.1042/bj3500589, 2000.
Pan, Y., Deng, L., Wang, H., He, K., and Xia, Q.: Histidine-rich glycoprotein (HRGP): Pleiotropic and paradoxical effects on macrophage, tumor microenvironment, angiogenesis, and other physiological and pathological processes, Genes Dis., 9, 381–392, https://doi.org/10.1016/j.gendis.2020.07.015, 2020.
Roca, J., Perez-Patino, C., Barranco, I., Padilla, L. C., Martinez, E. A., Rodriguez-Martinez, H., and Parrilla, I.: Proteomics in fresh and preserved pig semen: Recent achievements and future challenges, Theriogenology, 150, 41–47, https://doi.org/10.1016/j.theriogenology.2020.01.066, 2020.
Schroeder, A. C., Schultz, R. M., Kopf, G. S., Taylor, F. R., Becker, R. B., and Eppig, J. J.: Fetuin inhibits zona pellucida hardening and conversion of ZP2 to ZP2f during spontaneous mouse oocyte maturation in vitro in the absence of serum, Biol. Reprod., 43, 891–897, https://doi.org/10.1095/biolreprod43.5.891, 1990.
Simjak, P., Cinkajzlova, A., Anderlova, K., Klouckova, J., Kratochvilova, H., Lacinova, Z., Kavalkova, P., Krejci, H., Mraz, M., Parizek, A., Krsek, M., and Haluzik, M.: Changes in plasma concentrations and mRNA expression of hepatokines fetuin A, fetuin B and FGF21 in physiological pregnancy and gestational diabetes mellitus, Physiol. Res., 67, S531–S542, https://doi.org/10.33549/physiolres.934017, 2018.
Stocker, W., Karmilin, K., Hildebrand, A., Westphal, H., Yiallouros, I., Weiskirchen, R., Dietzel, E., Floehr, J., and Jahnen-Dechent, W.: Mammalian gamete fusion depends on the inhibition of ovastacin by fetuin-B, J. Biol. Chem., 395, 1195–1199, https://doi.org/10.1515/hsz-2014-0189, 2014.
Tomonobu, N., Kinoshita, R., Wake, H., Inoue, Y., Ruma, I. M. W., Suzawa, K., Gohara, Y., Komalasari, N. L. G. Y., Jiang, F., Murata, H., Yamamoto, K. I., Sumardika, I. W., Chen, Y., Futami, J., Yamauchi, A., Kuribayashi, F., Kondo, E., Toyooka, S., Nishibori, M., and Sakaguchi, M.: Histidine-rich glycoprotein suppresses the S100A8/A9-mediated organotropic metastasis of melanoma cells, Int. J. Mol. Sci, 23, 10300, https://doi.org/10.3390/ijms231810300, 2022.
Tsuchida-Straeten, N., Ensslen, S., Schafer, C., Woltjeo, M., Denecke, B., Moser, M., Graber, S., Wakabayashi, S., Koide, T., and Jahnen-Dechent, W.: Enhanced blood coagulation and fibrinolysis in mice lacking histidine-rich glycoprotein (HRG), J. Thromb. Haemost., 3, 865–872, https://doi.org/10.1111/j.1538-7836.2005.01238.x, 2005.
Tugues, S., Honjo, S., Konig, C., Noguer, O., Hedlund, M., Botling, J., Deschoemaeker, S., Wenes, M., Rolny, C., Jahnen-Dechent, W., Mazzone, M., and Claesson-Welsh, L.: Genetic deficiency in plasma protein HRG enhances tumor growth and metastasis by exacerbating immune escape and vessel abnormalization, Cancer Res., 72, 1953–1963, https://doi.org/10.1158/0008-5472.CAN-11-2194, 2012.
UniProt Consortium: Activities at the Universal Protein Resource (UniProt), Nucleic Acids Res, 42, D191-198, https://doi.org/10.1093/nar/gkt1140, 2014.
Wakabayashi, S.: New insights into the functions of histidine-rich glycoprotein, Int. Rev. Cel. Mol. Bio., 304, 467–493, https://doi.org/10.1016/B978-0-12-407696-9.00009-9, 2013.
Zhang, R., Cheng, F., Cheng, W., Wang, X., Zhang, B., Tian, M., Li, K., and Liu, D.: The Relationships among Plasma Fetuin-B, Thyroid Autoimmunity, and Fertilization Rate In Vitro Fertilization and Embryo Transfer, Int. J. Endocrinol, 2022, 9961253, https://doi.org/10.1155/2022/9961253, 2022.
Zhang, S., Tong, X., Zhang, Y., and Sun, X.: Early warning based on follicular fluid histidine-rich glycoprotein, C4b binding protein expression level as unexplained recurrent abortion, comprises e.g. collecting follicular fluid, centrifuging, taking supernatant, packaging, freezing, testing follicular fluid, counting and analyzing result, CN113791224-A, https://webofscience.clarivate.cn/wos/alldb/full-record/DIIDW:2022215010 (last access: 1 April 2024), 2021.
Zhu, K., Wang, Y., Shu, P., Zhou, Q., Zhu, J., Zhou, W., Du, C., Xu, C., Liu, X., and Tang, L.: Increased serum levels of fetuin B in patients with coronary artery disease, Endocr. Rev., 58, 97–105, https://doi.org/10.1007/s12020-017-1387-1, 2017.
Zhu, W., Zhang, Y., Ren, C.H., Cheng, X., Chen, J. H., Ge, Z. Y., Sun, Z. P., Zhuo, X., Sun, F. F., Chen, Y. L., Jia, X. J., and Zhang, Z.: Identification of proteomic markers for ram spermatozoa motility using a tandem mass tag (TMT) approach, J. Proteomics, 210, 103438, https://doi.org/10.1016/j.jprot.2019.103438, 2020.