the Creative Commons Attribution 4.0 License.
the Creative Commons Attribution 4.0 License.
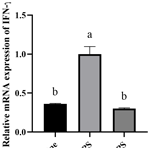
Capsanthin supplementation modulates the immune response in broiler chickens under Escherichia coli lipopolysaccharide challenge
Brigitta Csernus
Csaba Szabó
Renáta Knop
Reda Gebrehaweria Kidane
Sawadi Fransisco Ndunguru
Gabriella Gulyás
Xénia Erika Ozsváth
Levente Czeglédi
Due to the legislation of antibiotic usage, natural substances are required for application in the poultry industry. Because of their potential anti-inflammatory and immunomodulatory effects, carotenoids are great sources. Capsanthin, a major carotenoid giving the red color of pepper, is a promising feed additive, as it can reduce chronic inflammation. This study was conducted to determine the effects of capsanthin supplementation at 80 mg kg−1 in feed on the immune response of broiler chickens under Escherichia coli O55:B5 lipopolysaccharide (LPS) challenge. Ross 308 male broilers were divided into treatments: control (basal diet) and feed-supplemented groups. At 42 d of age, chickens were weighed and then challenged with 1 mg LPS per kilogram of body weight intraperitoneally. Four hours after injection, birds were euthanized, and then spleen and blood samples were collected. Capsanthin supplement at 80 mg kg−1 did not change the growth parameters and the relative spleen weight. LPS immunization resulted in higher splenic interleukin-1β (IL-1β), interleukin-6 (IL-6), and interferon-γ (IFN-γ) mRNA expressions. Capsanthin addition reached lower gene expression levels of IL-6 and IFN-γ compared to the LPS-injected birds. At plasma level, dietary capsanthin resulted in lower IL-1β and IL-6 levels. These results may indicate the potential anti-inflammatory effect of capsanthin supplementation in broiler chickens.
- Article
(1406 KB) - Full-text XML
- BibTeX
- EndNote
Inadequate usage of antibiotics in poultry is receiving great attention due to the potential residues in chicken meat and antimicrobial resistance among bacterial populations (Abd El-Hack et al., 2020). Accordingly, the application of antibiotics is being regulated, and natural substances such as plant extracts have appeared in recent years (Alagawany et al., 2018). Among the plant substances, carotenoids are often used in poultry feed as a means of pigmentation of animal products, last but not least for their anti-inflammatory, antioxidant, and immunomodulatory effects (Marounek and Pebriansyah, 2018; Nabi et al., 2020). The anti-inflammatory effects of some carotenoid compounds, such as astaxanthin or other xanthophylls (lutein, zeaxanthin), have been proven (Lee et al., 2003; Gao et al., 2012). However, other potential carotenoid agents can be applied in feed to improve immune responses during inflammatory reactions. Capsanthin is one of the major carotenoids of red pepper. It comprises up to 60 % of the total carotenoids in pepper, but the proportion can vary among cultivars in the genus Capsicum (Perez-Galvez et al., 2003; Suzuki-Mori, 2003). Capsanthin is fat-soluble and has a molecular structure with a long chain of conjugated double bonds ending in one or two polar ketones. It absorbs green light to give a red–orange shade (Shah et al., 2014). The mentioned compound has an important role in animal nutrition, health, and reproduction, and it can ameliorate chronic inflammation and has a higher antioxidant activity than other xanthophylls (Shah et al., 2014; Perez-Galvez and Minguez-Mosquera, 2002). It could reduce the oxidative stress and the inflammation and inhibit the expressions of inflammatory cytokines, tumor necrosis factor-α (TNF-α), pro-inflammatory mediators, and interleukins (IL-2, IL-4, IL-6) in rats (Shanmugham and Subban, 2022). Due to the very limited studies with capsanthin in birds, we aimed to investigate the effect of capsanthin on growth performance, relative spleen weight, and immune-related gene expression in broiler chickens under lipopolysaccharide (LPS) challenge. LPS is an integral component of the outer membrane of Gram-negative bacteria and causes acute or systemic inflammation (Akira et al., 2001; Guo et al., 2022). Therefore, it is a widely used model to study stress or inflammatory responses in broiler chickens (Lee et al., 2017; Chen et al., 2018). LPS can increase the degree of lipid peroxidation by elevating malondialdehyde (MDA) levels and decreasing the concentration of superoxide dismutase (SOD) antioxidant enzymes in male broilers at 42 d of age. In addition, LPS can enhance the gene expression levels of pro-inflammatory cytokines, such as interleukin-1β and interferon-γ at the same age of male chickens (Zhang et al., 2021). Among the immune-related genes, interleukin-1β (IL-1β), interleukin-6 (IL-6), interferon-γ (IFN-γ), and toll-like receptor 4 (TLR-4) were examined in our study. As part of the innate and adaptive immunity, cytokines have signaling roles between cells and take part in cellular immune responses. Both interleukins (IL-1β, IL-6) are pro-inflammatory ones (Dinarello, 2000; Kambayashi et al., 1995). IL-1β stimulates macrophages and has a role in inflammatory reactions, and it activates T cells (Lotz et al., 1988; Klasing, 1988), while the higher level of IL-6 can be attributed to an acute-phase reaction (Hong et al., 2006). IFN-γ is a multifunctional pro-inflammatory cytokine originally known to interfere with viral replication; however, it has several roles during immune responses, such as stimulating the bactericidal activity of phagocytes (Oladele et al., 2018). TLR-4 is a transmembrane protein that recognizes the presence of LPS (Akira et al, 2001). In this study, IL-1β and IL-6 cytokines were investigated at plasma protein level and, being part of the humoral immune response, immunoglobulin G (IgG) was also examined (Kaiser and Stäheli, 2008).
2.1 Experimental design and growth parameters
Ross 308 male broilers were hatched at a local hatchery (Master Good Ltd., Petnehaza, Hungary), and a trial was conducted at the University of Debrecen, Institute for Agricultural Research and Educational Farm, Animal Husbandry Experimental Station (Kismacs, Debrecen, Hungary). All broilers were placed in the same room and kept in cages covered by card boxes individually. Temperature and light were provided according to the Aviagen Ross Management Handbook. Broiler chickens were randomly divided into treatments. The experiment started at 35 d of age and lasted until 42 d of age, since physiological, stress, or immunological parameters are often studied at this latter age (Liu et al., 2015; Zheng et al., 2020). The experimental diets consisted of the control group (basal diet) and capsanthin supplementation at 80 mg kg−1 in feed. This is the maximum dosage at which capsanthin can be used (alone or in combination with other carotenoids or xanthophylls) as a colorant in poultry feed (except turkey) without any time limit established by the European Union Register of Feed Additives pursuant to Regulation (EC) No. 1831/2003 of Council Directive 70/524/EEC (European Food Safety Authority, 2020). Capsanthin was commercially available (ab142638, Abcam, Cambridge, UK) and dissolved in sunflower oil and then sprayed onto the basal diet during mixing. The basal diets (finisher) (Table 1) were corn–soybean meal diets and fed in mashed form. Broilers had free access to feed and water. On the last day of the trial, broiler chickens 42 d of age were weighed, and then daily gain and feed intake were determined. On the same day, six male broilers per treatment were injected with 1 mg kg−1 body weight Escherichia coli O55:B5 LPS (L2880, Sigma, St. Louis, MO, USA) intraperitoneally (Takahashi et al., 2013). LPS was dissolved in sterile isotonic saline solution (B. Braun, Budapest, Hungary) to provide a concentration of 1 mg mL−1. Another six male chickens in the control group were also inoculated with 1 mL kg−1 body weight saline solution (as the LPS vehicle in equivalent volume) in the same way.
2.2 Sample collection and lymphoid organ weight
Four hours later all of the injected birds were sacrificed by cervical dislocation. Blood was collected and separated into plasma by centrifugation at 3000 RCF (relative centrifugal force) and for 10 min. The whole spleen was aseptically excised and measured and then transferred to liquid nitrogen and stored at −80 ∘C for RNA isolation. The relative spleen weight was calculated as follows: spleen weight divided by live weight and multiplied by 100 (Sławińska et al., 2014).
2.3 Gene expression measurement
Isolation of total RNA was carried out from spleen samples using DirectZol™ RNA MiniPrep (Zymo Research, Orange, CA, USA) according to the manufacturer's protocol. The yield of the obtained RNA was calculated by applying the Biotek Synergy HTX Multimode Reader (Agilent Technologies, Inc., Santa Clara, CA, USA). RNA integrity was checked by 1 % agarose gel electrophoresis. The cDNA synthesis was performed from 200 ng of total RNA using qScript® cDNA supermix (Quantabio, Beverly, MA, USA) according to the manufacturer's protocol. cDNA samples were diluted 10-fold and stored at −20 ∘C. Primer pairs for chicken target genes (IL-1β, IL-6, IFN-γ, and TLR-4) were designed as reported in our previous study (Csernus et al., 2020). The primer details are listed in Table 2. Real-time PCR was carried out in an Agilent AriaMx real-time PCR (Agilent Technologies, Inc., Santa Clara, CA, USA). Reactions were run in duplicates using a 96-well plate (FrameStar, 4titude, Surrey, UK). Each reaction included 2 ng cDNA, 5× HOT FIREPol® EvaGreen® qPCR Mix Plus (ROX) (Solis BioDyne, Tartu, Estonia), and 200 nM of each primer and distilled water in a 10 µL final volume. No template controls were involved for each gene. RT(real-time)-PCR conditions were the following: initial denaturation at 90 ∘C for 12 min, 40 cycles of denaturation at 90 ∘C for 2 min, primer annealing at 60 ∘C for 20 s, and elongation at 72 ∘C for 20 s. Ct values and melting curves were collected by AriaMx 1.8 software. In the spleen, GAPDH was considered the most stable gene for normalization by the NormFinder, Best Keeper, and ΔCt methods. Results were calculated using the 2ΔΔCt relative quantitative method (Livak and Schmittgen, 2001). Relative expressions were determined as fold changes in the expression of the target gene in the treatment group compared with the LPS-injected control group.
2.4 Enzyme-linked immunosorbent assay (ELISA)
Chicken plasma samples were used to evaluate IL-1β, IL-6, and IgG levels with an ELISA kit (Xinqidi, Wuhan, China). ELISA kits followed the sandwich ELISA technique. Blanks, standards, and samples were involved in duplicates. Based on the kit specifications, the absorbance was determined at 450 nm using the Biotek Synergy HTX Multimode Reader (Agilent Technologies, Inc., Santa Clara, CA, USA). Protein concentrations were calculated using the equation from the linear regression of the obtained standard curve.
2.5 Statistical analysis
The main effect of capsanthin treatment under LPS challenge was analyzed using a one-way analysis of variance (one-way ANOVA) Tukey test by GraphPad Prism 8.0.1 software. Differences among treatments were considered significant at P<0.05. Results were presented as the mean ± standard error of the mean (SEM).
3.1 Growth parameters
Our results showed that capsanthin supplementation at a concentration of 80 mg kg−1 in feed did not affect the growth performance (Table 3) of broiler chickens (P>0.05).
3.2 Relative spleen weight and immune-related gene expression
Relative spleen weight did not show significant differences (P>0.05) among the groups (Fig. 1). Results were 0.108 % in control (saline) birds, 0.123 % in the control (LPS) group, and 0.114 % in the capsanthin (LPS) treatment.
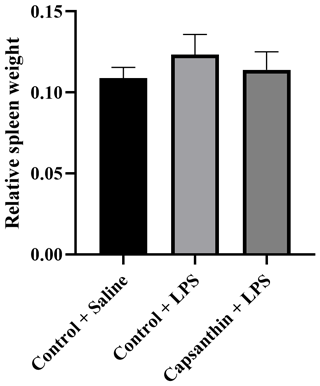
Figure 1Relative spleen weight of broiler chickens. Control + saline: chickens fed a basal diet with isotonic saline injection. Control + LPS: chickens fed a basal diet under E. coli O55:B5 LPS challenge. Capsanthin + LPS: chickens fed a diet supplemented with capsanthin at 80 mg kg−1 under E. coli O55:B5 LPS challenge ( treatment). Data are presented as means ± standard errors of the mean. The impact was analyzed by one-way ANOVA, and differences among the groups were considered significant at P<0.05. The effect of dietary supplementation was not significant.
Relative mRNA expressions of IL-1β, IL-6, IFN-γ, and TLR-4 are shown in Fig. 2. The gene expression level of the pro-inflammatory IL-1β was higher (P=0.0002) in the control (LPS) group compared to the saline-injected control group. Capsanthin supplementation at 80 mg kg−1 in feed did not result in a lower gene expression level of IL-1β compared to the LPS-injected birds. The gene expression level of the pro-inflammatory IL-6 was also higher (P<0.0001) in the LPS-injected control birds compared to the control (saline) ones. Capsanthin addition at 80 mg kg−1 decreased the level of IL-6 compared to the LPS group. The relative mRNA expression of IFN-γ was higher in the LPS-treated birds, and capsanthin supplementation eventuated in a lower (P=0.0382) mRNA level of the pro-inflammatory IFN-γ. Relative mRNA levels of TLR-4 were lower in the control (LPS) (P=0.0351) and capsanthin (LPS) (P=0.0023) groups compared to control (saline) birds.
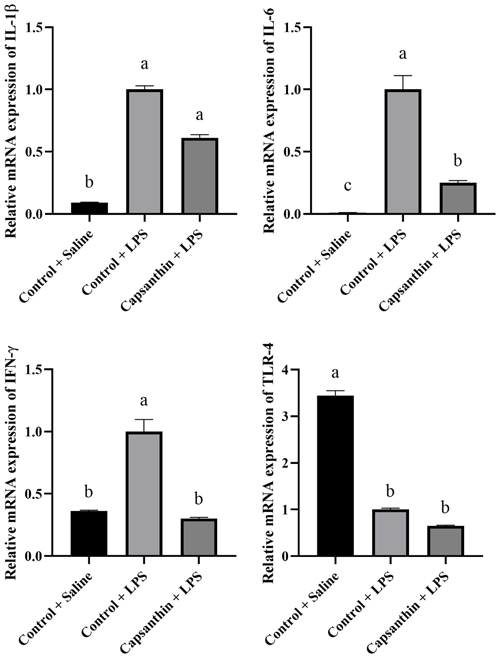
Figure 2Relative mRNA levels of splenic interleukin-1β (IL-1β), interleukin-6 (IL-6), interferon-γ (IFN-γ), and toll-like receptor 4 (TLR-4) in broiler chickens. Control + saline: chickens fed a basal diet with isotonic saline injection. Control + LPS: chickens fed a basal diet under E. coli O55:B5 LPS challenge. Capsanthin + LPS: chickens fed a diet supplemented with capsanthin at 80 mg kg−1 under E. coli O55:B5 LPS challenge ( treatment). Data are presented as means ± standard errors of the mean. The impact was analyzed by one-way ANOVA, and differences among the groups were considered significant at P<0.05. Means with a, b, and c differ significantly at P<0.05.
3.3 Plasma IL-1β, IL-6, and IgG concentrations
Plasma concentrations of IL-1β, IL-6, and IgG are shown in Fig. 3. IL-1β levels were 32.19, 25.62, and 11.24 pg mL−1 in the control (saline), control (LPS), and capsanthin (LPS) groups, respectively. Concentrations of IL-1β did not differ among the control (LPS) and control (saline) groups. Capsanthin supplementation decreased (P=0.0496) the level of the mentioned pro-inflammatory cytokine compared to the LPS-injected birds. The concentration of pro-inflammatory IL-6 was 121.2, 137.8, and 85.79 pg mL−1 in the control (saline), control (LPS), and capsanthin (LPS) groups, respectively. IL-6 concentrations did not differ significantly between the control groups (LPS and saline). However, capsanthin supplementation could reach a lower level (P=0.0263) of IL-6 compared to LPS-injected chickens. Plasma IgG concentrations were 203.3, 237.2, and 209.7 ng mL−1 in the control (saline), control (LPS), and capsanthin (LPS) treatments, respectively. IgG levels did not differ significantly among the groups.
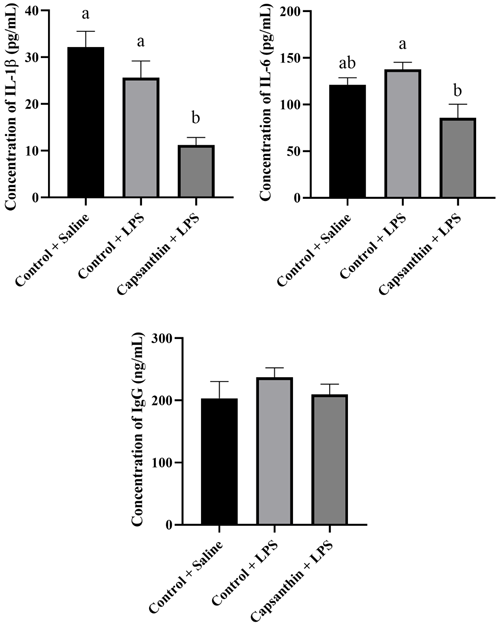
Figure 3Concentrations of plasma interleukin-1β (IL-1β), interleukin-6 (IL-6), and immunoglobulin G (IgG) of broiler chickens. Control + saline: chickens fed a basal diet with isotonic saline injection. Control + LPS: chickens fed a basal diet under E. coli O55:B5 LPS challenge. Capsanthin + LPS: chickens fed a diet supplemented with capsanthin at 80 mg kg−1 under E. coli O55:B5 LPS challenge ( treatment). Data are presented as means ± standard errors of the mean. The impact was analyzed by one-way ANOVA, and differences among the groups were considered significant at P<0.05. Means with a and b differ significantly at P<0.05.
A short experiment was conducted to investigate the effect of capsanthin supplementation on growth performance and immune response under Escherichia coli O55:B5 LPS challenge. The effect of feed supplemented with capsanthin at 80 mg kg−1 was examined on growth parameters, such as body weight, body weight gain, and feed intake. Due to the limited number of chickens ( control and treatment), performance parameters were calculated as background information to support the immunological study as the core part of the trial. None of the mentioned traits was affected by the supplementation. In contrast, chili pepper powder addition at 0.5 % and 1 % in the feed had positive effects on the body weight of chickens at 35 and 42 d of age (Puvača et al., 2019). Al-Kassie et al. (2011) reported the same, and body weight and feed conversion ratio were improved by chili pepper treatments at levels of 0.5 %, 0.75 %, and 1 % in the feed of broiler chickens. Thiamhirunsopit et al. (2014) also noted improved growth performance results after different chili pepper treatments compared to control birds.
The effect of capsanthin supplementation on immune response in broiler chickens under E. coli LPS challenge was also examined. LPS immunization induces an acute inflammatory response and stimulates the synthesis of pro-inflammatory cytokines in broilers. In this research, LPS inoculation was applied in both the capsanthin-treated and control groups to evoke immune response and to evaluate the impact of the mentioned carotenoid on immunological parameters during inflammatory responses. Saline inoculation was used as a vehicle in equivalent volume in the control group, and the impact of capsanthin was not examined without causing inflammation.
In our study, the relative spleen weights were not changed among the treatments. In contrast, the relative weight of this lymphoid organ was higher in the LPS-injected broilers compared to the lutein-supplemented, saline-injected birds (Rajput et al., 2013). Koutsos et al. (2006) reported higher spleen weights for the non-supplemented birds under LPS challenge, in contrast to the lutein-treated birds, and the authors discussed severe systemic inflammatory response after LPS injection for the non-treated birds. In this study, immune-related gene expression analysis was carried out to investigate the effect of capsanthin in broiler chickens under LPS immunization. The relative mRNA level of IL-1β was higher in the LPS-treated control group compared to the saline-injected control birds. Similarly, gene expression levels of splenic and ileal IL-1β were also increased in the LPS-injected control birds (Wu et al., 2017). Therefore, E. coli lipopolysaccharides induced an acute-phase response and a bacterial illness, which was also confirmed in our previous study (Csernus et al., 2020). Capsanthin supplementation decreased the mRNA expression of the mentioned interleukin. Similarly, splenic IL-1β was decreased in chickens by dried-scent leaf meal (rich in carotenoids), and the authors discussed that the dried-scent leaf meal may regulate the inflammation in chickens and may be used as a replacement for in-feed antibiotics in chicken production (Sorhue et al., 2021).
In our study, the gene expression level of pro-inflammatory IL-6 was elevated in the LPS-injected birds in contrast to the saline-inoculated ones, which can be discussed as an acute-phase reaction (Hong et al., 2006). Capsanthin supplementation reduced the mRNA level of the cytokine. Meriwether et al. (2010) reported the same, and splenic IL-6 mRNA abundance was higher in the LPS-injected laying chickens, in contrast to the control (non-vaccinated) ones. The authors also applied lutein (a carotenoid source) at a concentration of 40 mg kg−1 in the diet of laying chicks through 30 d, resulting in carotenoid-replete eggs. Twelve days after hatching, an inflammatory challenge (LPS) was used. As a result of in ovo carotenoid exposure, the mRNA level of IL-6 could decrease compared to those chickens hatched from carotenoid-deplete eggs, and as discussed, lutein can decrease the inflammatory parameters in the spleen (Meriwether et al., 2010).
In this study, capsanthin influenced the level of pro-inflammatory IFN-γ as well, and the supplement at 80 mg kg−1 reached a lower gene expression level of the mentioned interferon. Similarly, the relative mRNA level of IFN-γ was inhibited in the liver and jejunum of chickens without any challenge on experimental day 35, when dietary xanthophylls were fed at 40 mg kg−1 (Gao et al., 2012). Pourabedin et al. (2017) noted the same, and feed supplementation reduced the level of IFN-γ cecal tonsils of chickens under Salmonella enteritidis challenge. In the same study, the authors concluded that IFN-γ takes part in macrophage activation and nitric oxide production.
TLR-4 is an important receptor of LPS and stimulates the secretion of pro-inflammatory cytokines, which are crucial for enhancing potent immune responses (Gorina et al., 2011; Mateu et al., 2015). In our study, the relative mRNA expression level of TLR-4 decreased in the LPS-challenged control group. Since TLR-4 recognizes LPS, the opposite was expected. Similarly to our results, Guo et al. (2022) noted the same, and the gene expression level of TLR-4 was lower in the LPS-injected broiler chickens when LPS was applied at the same concentration (1 mg kg−1 BW). The authors discussed that chickens may respond differently to LPS compared to mammals, and the responding patterns may be attributed to the diverse species and LPS dosages. The gene expression level of TLR-4 was inhibited in capsanthin-fed birds as well. Cheng et al. (2017) reported the same when baicalin (a flavonoid compound) at concentrations of 50, 100, and 200 mg kg−1 in feed decreased the gene expression levels of TLR-4 and concluded that the flavonoid could alleviate LPS-induced inflammatory responses in chickens and have an anti-inflammatory effect.
The impact of capsanthin supplementation on plasma concentrations of IL-1β, IL-6, and IgG was also determined in this study. In contrast to the relative mRNA expressions in spleen, plasma protein levels of IL-1β and IL-6 did not change in the LPS-injected control group compared to the saline-inoculated control birds, which could be due to the post-transcriptional phenomena, such as the mRNA stability or the elevated half life of protein resulting from the post-translational modifications often altering the protein levels (Ideker et al., 2001). However, capsanthin supplementation decreased plasma IL-1β concentration compared to both control groups, which may indicate the potential anti-inflammatory property of the mentioned carotenoid. The plasma concentration of IL-6 was lower in the feed-supplemented group compared to the LPS-injected control birds, so capsanthin may decrease the level of inflammation at plasma level as well. The effect of capsanthin on plasma IgG concentration was also examined in this study; however, it did not change in the treated group. Zhou et al (2019) reported that feed supplementation resulted in elevated levels of jejunal and ileal IgG contents, and the authors discussed improved immune function. Cai et al. (2012) defined increased serum IgG levels in broiler chickens when feed supplementation was applied, which was explained with improved humoral immunity by the authors.
In conclusion, dietary capsanthin at the applied concentration could mostly affect the immune parameters of broiler chickens under Escherichia coli LPS challenge. Capsanthin supplementation at 80 mg kg−1 in feed decreased the relative gene expression levels of IL-6 and IFN-γ and further reduced plasma IL-1β and IL-6 concentrations compared to the LPS-injected birds. Therefore, the usage of capsanthin is being suggested to mitigate the inflammation during cellular immune response in broiler chickens.
The original data of the paper are available upon request from the corresponding author.
BC, CS, and LC conceived and designed the experiments. BC, RK, RGK, SFN, and XEO performed the experiments. BC and LC analyzed the data. CS, RK and LC contributed materials. BC, GG, and LC wrote and revised the paper.
The contact author has declared that none of the authors has any competing interests.
Experiments were confirmed by the University of Debrecen Committee of Animal Welfare, Hungary (permit number 4/2021/DEMAB).
Publisher’s note: Copernicus Publications remains neutral with regard to jurisdictional claims in published maps and institutional affiliations.
Project no. TKP2020-NKA-04 has been implemented with the support provided by the National Research, Development, and Innovation Office of Hungary financed under the 2020-4.1.1-TKP2020 funding scheme. Brigitta Csernus appreciates the support of the OTKA grant (K139021) and is grateful for the contribution of the New National Excellence Program (ÚNKP-20-4-I-DE-101) of the Ministry of Human Capacities.
This research has been supported by the National Research, Development and Innovation Office (grant no. TKP2020-NKA-04), the Hungarian Scientific Research Fund (grant no. K139021), and the Ministry of Human Capacities (grant no. ÚNKP-20-4-I-DE-101).
This paper was edited by Manfred Mielenz and reviewed by Elİf Babacanoğlu and one anonymous referee.
Abd El-Hack, M. E., Alagawany, M., Shaheen, H., Samak, D., Othman, S. I., Allam, A. A., Taha, A. E., Khafaga, A. F., Arif, M., Osman, A., Sheikh, A. I., Elnesr, S. S., and Sitohy, M.: Ginger and Its Derivatives as Promising Alternatives to Antibiotics in Poultry Feed, MDPI Animals, 10, 452, https://doi.org/10.3390/ani10030452, 2020.
Akira, S., Takeda, K., and Kaisho, T.: Toll-like receptors: Critical proteins linking innate and acquired immunity, Nat. Immunol., 2, 675–680, https://doi.org/10.1038/90609, 2001.
Alagawany, M., Abd El-Hack, M. E., Farag, M. R., Sachan, S., Karthik, K., and Khama, K.: The use of probiotics as eco-friendly alternatives for antibiotics in poultry nutrition, Environ. Sci. Pollut. Res., 25, 10611–10618, https://doi.org/10.1007/s11356-018-1687-x, 2018.
Al-Kassie, G. A. M., Al-Nasrawi, M. A. M., and Ajeena, S. J.: The effects of using hot red pepper as a diet supplement on some performance traits in broiler, Pak. J. Nutr., 10, 842–845, https://doi.org/10.3923/pjn.2011.842.845, 2011.
Cai, S. J., Wu, C. X., Gong, L. M., Song, T., Wu, H., and Zhang, L. Y.: Effects of nanoselenium on performance, meat quality, immune function, oxidation resistance, and tissue selenium content in broilers, Poult. Sci., 91, 2532–2539, https://doi.org/10.3382/ps.2012-02160, 2012.
Chen, Y., Zhang, H., Cheng, Y., Li, Y., Wen, C., and Zhou, Y.: Dietary L-threonine supplementation attenuates lipopolysaccharide-induced inflammatory responses and intestinal barrier damage of broiler chickens at an early age, Brit. J. Nutr., 119, 1254–1262, https://doi.org/10.1017/S0007114518000740, 2018.
Cheng, P., Wang, T., Li, W., Muhammad, I., Wang, H., Sun, X., Yang, Y., Li, J., Xiao, T., and Zhang, X.: Baicalin Alleviates Lipopolysaccharide-Induced Liver Inflammation in Chicken by Suppressing TLR4-Mediated NF-κB Pathway, Front. Pharmacol., 8, 547, https://doi.org/10.3389/fphar.2017.00547, 2017.
Csernus, B., Biró, S., Babinszky, L., Komlósi, I., Jávor, A., Stündl, L., Remenyik, J., Bai, P., Oláh, J., Pesti-Asbóth, G., and Czeglédi, L.: Effect of Carotenoids, Oligosaccharides and Anthocyanins on Growth Performance, Immunological Parameters and Intestinal Morphology in Broiler Chickens Challenged with Escherichia coli Lipopolysaccharide, Animals, 10, 347, https://doi.org/10.3390/ani10020347, 2020.
Dinarello, C. A.: Proinflammatory cytokines, Chest, 118, 503–508, 2000.
European Food Safety Authority: Safety and efficacy of saponified paprika extract, containingcapsanthin as main carotenoid source, for poultry forfattening and laying (except turkeys), EFSA J., 18, 6023, https://doi.org/10.2903/j.efsa.2020.6023, 2020.
Gao, Y.-Y., Xie, Q.-M., Jin, L., Sun, B. L., Ji, J., Chen, F., Ma, J.-Y., and Bi, Y.-Z.: Supplementation of xanthophylls decreased proinflammatory and increased anti-inflammatory cytokines in hens and chicks, Brit. J. Nutr., 108, 1746–1755, https://doi.org/10.1017/S0007114512000025, 2012.
Gorina, R., Font-Nieves, M., Marquez-Kisinousky, L., Santalucia, T., and Planas, A. M.: Astrocyte TLR4 activation induces a proinflammatory environment through the interplay between MyD88-dependent NFkappaB signaling, MAPK, and Jak1/Stat1 pathways, Glia, 59, 242–255, https://doi.org/10.1002/glia.21094, 2011.
Guo, F., Jing, M., Zhang, A., Yu, Y., Gao, P, Wang, Q., Wang, L., Xu, Z., Ma, J., and Zhang, Y.: Betaine Alleviates LPS-Induced Chicken Skeletal Muscle Inflammation with the Epigenetic Modulation of the TLR4 Gene, MDPI Animals, 12, 1289, https://doi.org/10.3390/ani12151899, 2022.
Hong, Y. H., Lillehoj, H. S., Lillehoj, E. P., and Lee, S. H.: Changes in immune-related gene expression and intestinal lymphocyte subpopulations following Eimeria maxima infection in chickens, Vet. Immunol. Immunopathol., 114, 259–272, https://doi.org/10.1016/j.vetimm.2006.08.006, 2006.
Ideker, T., Thorsson, V., Ranish, J.A., Christmas, R., Buhler, J., Eng, J. K., Bumgarner, R., Goodlett, D. R., Aebersold, R., and Hood L.: Integrated genomic and proteomic analyses of a systematically perturbed metabolic network, Science, 292, 929–934, https://doi.org/10.1126/science.292.5518.929, 2001.
Kaiser, P. and Stäheli, P.: Avian cytokines and chemokines, in: Avian Immunol; edited by: Davison, F., Kaspers, B., and Schat, K. A., Elsevier, London, UK, Academic Press, p. 203, ISBN: 978-0-12-396965-1, 2008.
Kambayashi, T., Jacob, C.O., Zhou, D., Mazurek, N., Fong, M., and Strassmann, G.: Cyclic nucleotide phosphodiesterase type IV participates in the regulation of IL-10 and in the subsequent inhibition of TNF-alpha and IL-6 release by endotoxin-stimulated macrophages, J. Immun., 155, 4909–4916, 1995.
Klasing, K. C.: Nutritional aspects of leukocytic cytokine cytokines, J. Nutr., 118, 1436–1446, https://doi.org/10.1093/jn/118.12.1436, 1988.
Koutsos, E. A., García Lopez, J. C., and Klasing, K. C.: Carotenoids from in ovo or dietary sources blunt systemic indices of the inflammatory response in growing chicks (Gallus gallus domesticus), J. Nutr., 136, 1027–1031, https://doi.org/10.1093/jn/136.4.1027, 2006.
Lee, S. J., Bai, S. K., and Lee, K. S.: Astaxanthin inhibits nitric oxide production and inflammatory gene expression by suppressing I(kappa)B kinase-dependent NF-kappaB activation, Mol. Cells, 16, 97–105, 2003.
Lee, Y., Lee, S.-H., Gadde, U.-D., Oh, S.-T., Lee, S.-J., and Lillehoj, H.-S.: Dietary Allium hookeri reduces inflammatory response and increases expression of intestinal tight junction proteins in LPS-induced young broiler chicken, Res. Vet. Sci., 112, 149–155, https://doi.org/10.1016/j.rvsc.2017.03.019, 2017.
Liu, L., Qin, D., Wang, X., Feng, Y., Yang, X., and Yao, J.: Effect of immune stress on growth performance and energymetabolism in broiler chickens, Food Agr. Immunol., 26, 194–203, https://doi.org/10.1080/09540105.2014.882884, 2015.
Livak, K. J. and Schmittgen, T. D.: Analysis of relative gene expression data using real-time quantitative PCR and the 2(-Delta Delta C(T)) Method, Methods, 22, 405–408, https://doi.org/10.1006/meth.2001.1262, 2001.
Lotz, M., Jirik, F., Kabouridis, P., Tsoukas, P., Hirano, T., Kishimoto, T., and Carson, D. A.: B cell stimulating factor 2/Interleukin 6 is a co-stimulant for human thymocytes and T lymphocytes, J. Exp. Med., 167, 1253–1268, https://doi.org/10.1084/jem.167.3.1253, 1988.
Marounek, M. and Pebryansyah, A.: Use of carotenoids in feed mixtures for poultry: a review, Agricultura Tropica et Subtropica, 51, 107–111, https://doi.org/10.2478/ats-2018-0011, 2018.
Mateu, A., Ramudo, L., Manso, M. A., and De Dios, I.: Cross-talk between TLR4 and PPARgamma pathways in the arachidonic acid-induced inflammatory response in pancreatic acini, Int. J. Biochem. Cell Biol., 69, 132–141, https://doi.org/10.1016/j.biocel.2015.10.022, 2015.
Meriwether, L. S., Humphrey, B. D., Peterson, D. G., Klasing, K. C., and Koutsos, E. A.: Lutein exposure, in ovo or in the diet, reduces parameter of inflammation in the liver and spleen laying-type chicks (Gallus gallus domesticus), J. Anim. Physiol. Anim. Nutr., 94, 115–122, https://doi.org/10.1111/j.1439-0396.2010.00990.x, 2010.
Nabi, F., Arain, M. A., Rajput, N., Alagawany, M., Soomro, J., Umer, M., Soomro, F., Wang, Z., Ye, R., and Liu, J.: Health benefits of carotenoids and potential application in poultry industry: A review, J. Anim. Physiol. Anim. Nutr., 104, 1809–1818, https://doi.org/10.1111/jpn.13375.
Oladele, O. A., Esan, O. O., Adetiba, I. A., Akpan, I. L., and Adewale, O. M.: Haematological, Serum Biochemical and Immunological Changes Associated with the Inclusion of Garlic (Allium sativum L.) Meal in the Diet of Commercial Layer Chickens, J. Poult. Res., 15, 8–15, 2018.
Perez-Galvez, A. and Minguez-Mosquera, M. I.: Degradation of non-esterified and esterified xanthophylls by free radicals, Biochim. Biophys. Ac., 1569, 31–34, https://doi.org/10.1016/s0304-4165(01)00229-x, 2002.
Perez-Galvez, A., Martin, H. D., Sies, H., and Stahl, W.: Incorporation of carotenoids from paprika oleoresin into human chylomicrons, Br. J. Nutr., 89, 787–93, https://doi.org/10.1079/BJN2003842, 2003.
Pourabedin, M., Chen, Q., Yang, M., and Zhao, X.: Mannan- and xylooligosaccharides modulatecaecal microbiota and expression of inflammatory-related cytokines and reduce caecal Salmonella enteritidis colonization in young chickens, FEMS Microbiol. Ecol., 93, 1–11, https://doi.org/10.1093/femsec/fiw226, 2017.
Puvača, N., Pelić, D. L., Popović, S., Ikonić, P., Đuragić, O., Peulić, T., and Lević, J.: Evaluation of broiler chickens lipid profile influenced by dietary chili pepper addition, J. Agr., 2, 318–324, 2019.
Rajput, N., Naeem, M., Ali, S., Zhang, J. F., Zhang, L., and Wang, T.: The effect of dietary supplementation with the natural carotenoids curcumin and lutein on broiler pigmentation and immunity, Poult. Sci., 92, 1177–1185, https://doi.org/10.3382/ps.2012-02853, 2013.
Shah, S. N. M., Tian, S.-L., Gong, Z.-H., and Arisha, M. H.: Studies on Metabolism of Capsanthin and Its Regulation under Different Conditions in Pepper Fruits (Capsicum spp.), Ann. Res. Rev. Biol., 4, 1106–1120, https://doi.org/10.9734/ARRB/2014/6542, 2014.
Shanmugham, V. and Subban, R.: Capsanthin from Capsicum annum fruits exerts anti-glaucoma, antioxidant, anti-inflammatory activity, and corneal pro-inflammatory cytokine gene expression in a benzalkonium chloride-induced rat dry eye model, J. Food Biochem., 46, e14352, https://doi.org/10.1111/jfbc.14352, 2022.
Sławińska, A., Siwek, M., Zylińska, J., Bardowski, J., Brzezińska, J., Gulewicz, K. A., Nowak, M., Urbanowski, M., Płowiec, A., and Bednarczyk, M.: Influence of synbiotics delivered in ovo on immune organs development and structure, Folia Biol., 62, 277–285, https://doi.org/10.3409/fb62_3.277, 2014.
Sorhue, U. G., Onainor, E. R., Moemeka, A. M., and Peterson, I.-E. E.: Dietary Inclusion of Scent Leaf Meal (Ocimum Gratissimum) Affects Immune Genes Expression in Chicken Spleen at 28 and 56 Days, Animal Rev., 8, 10–19, https://doi.org/10.18488/journal.ar.2021.81.10.19, 2021.
Suzuki, K. and Mori, M.: Carotenoid composition of new cultivar of Capsicum annuum during maturation and its high capsanthin content, J. Jpn. Socr. Food Sci. Tech., 50, 324–26, 2003.
Takahashi, K., Takimoto, T., Sato, K., and Akiba, Y.: Effect of dietary supplementation of astaxanthin from Phaffia rhodozyma on lipopolysaccharide-induced early inflammatory responses in male broiler chickens (Gallus gallus) fed a corn-enriched diet, Anim. Sci. J., 82, 753–758, https://doi.org/10.1111/j.1740-0929.2011.00898.x, 2011.
Thiamhirunsopit, K., Phisalaphong, C., Boonkird, S., and Kijparkorn, S.: Effect of chili meal (Capsicum frutescens LINN.) on growth performance, stress index, lipid peroxidation and ileal nutrient digestibility in broilers reared under high stocking density condition, Anim. Feed Sci. Technol., 192, 90–100, https://doi.org/10.1016/j.anifeedsci.2014.03.009, 2014.
Wu, Q. J., Wang, Y. Q., and QI, Y. X.: Influence of procyanidin supplementation on the immune responses of broilers challenged with lipopolysaccharide, Anim. Sci. J., 88, 983–990, https://doi.org/10.1111/asj.12729, 2017.
Zhang, C., Li, C. X., Shao, Q., Chen, W. B., Ma, L., Xu, W. H., Li, Y. X., Huang, S. C., and Ma, Y. B.: Effects of Glycyrrhiza polysaccharide in diet on growth performance, serum antioxidant capacity, and biochemistry of broilers, Poult. Sci., 100, 100927, https://doi.org/10.1016/j.psj.2020.12.025, 2021.
Zheng, Y. W., Zhang, J. Y., Zhou, H. B., Guo, Y. P., Ma, Q. G., Ji, C., and Zhao, L. H.: Effects of dietary pyrroloquinoline quinone disodium supplementation on inflammatory responses, oxidative stress, and intestinal morphology in broiler chickens challenged with lipopolysaccharide, Poult. Sci., 99, 5389–5398, https://doi.org/10.1016/j.psj.2020.08.007, 2020.
Zhou, M., Tao, Y., Lai, C., Huang, C., Zhou, Y., and Yong, Q.: Effects of Mannanoligosaccharide Supplementation on the Growth Performance, Immunity, and Oxidative Status of Partridge Shank Chickens, Animals, 9, 817, https://doi.org/10.3390/ani9100817, 2019.