the Creative Commons Attribution 4.0 License.
the Creative Commons Attribution 4.0 License.
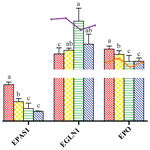
Characteristics of Tibetan pig lung tissue in response to a hypoxic environment on the Qinghai–Tibet Plateau
Yanan Yang
Caixia Gao
Tianliang Yang
Yuzhu Sha
Yuan Cai
Xinrong Wang
Qiaoli Yang
Chengze Liu
Biao Wang
To adapt to the plateau environment, Tibetan pigs' lungs have developed a unique physiological mechanism during evolution. The vascular corrosion casting technique and scanning electron microscopy were used to understand arterial architecture. Blood physiological index and quantitative real-time PCR (qRT-PCR) were used for assessing whether the lung can regulate the body through anatomical, physiological and molecular mechanisms to adapt to hypoxic environments. Our study showed that the lungs of Tibetan pigs were heavier and wider and that the pulmonary arteries were thicker and branched and had a denser vascular network than those of Landrace pigs. The hemoglobin (HGB), mean corpuscular hemoglobin concentration (MCHC) values of high-altitude pigs were significantly higher than those of low-altitude pigs. The expression levels of HIF-1α, EPAS1, EPO and VEGF, but not those of eNOS and EGLN1, were significantly higher in the lungs of high-altitude pigs than in those from pigs at a lower altitude (P<0.05). These findings and a comprehensive analysis help elucidate the pulmonary mechanism of hypoxic adaptation in pigs.
- Article
(1897 KB) -
Supplement
(471 KB) - BibTeX
- EndNote
-
Compared with Landrace pigs, Tibetan pigs had heavier and wider lungs, and their pulmonary arteries were thick, branched and had a denser vascular network than those of Landrace pigs.
-
Hemoglobin concentration (HGB), mean corpuscular hemoglobin concentration (MCHC) and hemoglobin content (Hb*) of high-altitude pigs (Tibetan pigs in Gannan, TGN, and Landrace pigs in Yongdeng, LYD) were significantly higher than those of low-altitude pigs (Tibetan pigs in Jingchuan, TJC, and Landrace pigs in Jingchuan, LJC).
-
The expression levels of HIF-1, EPAS1, EPO and VEGF, in contrast to eNOS and EGLN1, were significantly higher in the lungs of pigs at high altitude (TGN and LYD) than in those at low altitude (TJC and LJC) (P<0.05).
Hypoxia can trigger a series of stress reactions, such as metabolic disorders and tissue and cytomembrane damage (Jeong et al., 2018; Gan et al., 2019; Yanyu et al., 2019). To adapt to high-altitude environments, indigenous animals have developed unique physiological mechanisms during the long process of evolution and have acquired stable structural and functional characteristics to ensure a sufficient oxygen supply in their tissues and cells under hypoxic conditions (Ishikawa et al., 2019; Patra et al., 2019; Zhao et al., 2019). Tibetans have larger lungs and lower hemoglobin (HGB) concentrations than other groups, and the lungs of yaks and Tibetan sheep have larger pulmonary alveolar areas per unit area, thicker diameters, more branches and denser pulmonary artery networks than those of low-altitude animals (Wang et al., 2020; Moore et al., 2001; Qi et al., 2019). Physiological studies have shown that Tibetan pigs, as plateau mammals native to the Tibetan Plateau, have evolved physiological adaptations, such as increases in the size and strength of the heart and lung in response to high-altitude hypoxic conditions (Ai et al., 2014). Tibetan pigs have a thicker alveolar diaphragm and more capillaries than Landrace pigs (Bai et al., 2012). The red blood cell (RBC) number and HGB level of Tibetan pigs at high altitudes are significantly higher than those of low-altitude pigs (Kong et al., 2014).
Hypoxic adaptability is also reflected by the level of hypoxia-related gene regulation (Ishikawa et al., 2019; Yu et al., 2018; Bi et al., 2017; Cheng et al., 2016). The genes involved in the hypoxia-induced pathway include the positive regulatory factors, hypoxia-inducible factor (HIF-1α), vascular endothelial growth factor (VEGF), endothelial PAS domain-containing protein 1 (EPAS1), endothelial nitric oxide synthase (eNOS), erythropoietin (EPO) and the negative regulatory factor egl nine homolog 1 (EGLN1), and their regulated expression can lead to large increases in hypoxia-related substances (Ishikawa et al., 2019; Mohamed et al., 2019; Ma et al., 2019). At present, the mechanisms related to the adaptation of Tibetan pigs to high altitude have not been fully elucidated. The architecture of the pulmonary arteries of Tibetan pigs has rarely been studied. In this study, we collected pulmonary tissue samples from Tibetan pigs living at 3000 and 1000 m and Landrace pigs living at 2500 and 1000 m. The morphology of pulmonary artery cast specimens, the values of blood physiological indexes and the expression of hypoxia-responsive genes were compared to help elucidate the biological mechanism underlying the adaptation of Tibetan pigs to high-altitude hypoxic conditions.
2.1 Ethics statement
All animal experiments were conducted according to the guidelines for the care and use of experimental animals established by the Ministry of Science and Technology of the People's Republic of China (approval number: 2006-398), and the work was approved by Gansu Agricultural University, Lanzhou, China.
2.2 Sample collection
Forty-four fresh lungs from two breeds – Tibetan pigs that live in Gannan (TGN, altitude of 3000 m) and Jingchuan (TJC, altitude of 1000 m, moved from Gannan) and Landrace pigs that live in Yongdeng (LYD, altitude of 2500 m) and Jingchuan (LJC, altitude of 1000 m) in Gansu – were collected. We bred 12 male piglets from the population with a similar weight and non-genetic relationship for every group. We randomly selected three pigs from each group to collect the left lower lobes of the lung from adult male pigs at the age of 6 months within 1 h after the pigs were slaughtered, and the samples were stored immediately in liquid nitrogen for later RNA extraction; six lungs were cleaned to create three-dimensional architectures. Samples of venous blood were collected.
2.3 Generation of casts and measurement of the diameters
The arteries of the left lung were perfused with ABS (acrylonitrile butadiene styrene) agent using the following protocol: first, the blood of the lung was repeatedly squeezed from the margin to the aorta to expel congestion. Second, a needle was inserted and fixed from the left pulmonary artery, and the ABS agent (12 %) was injected. The sample was placed in cold water for 1 h and reperfused several times. The sample was then solidified and soaked in 31 % concentrated hydrochloric acid for 2 weeks to obtain a cast of the whole specimen. Photographs of the morphological specimens were obtained with a digital camera, and their anatomic characteristics were observed and compared among different breeds.
The diameters of the arteries and arterioles in the same parts of the lungs of Tibetan and Landrace pigs at different altitudes were measured and recorded using Vernier calipers, and the results were calculated as the average from three parts, namely, the root, middle and terminal sections. Microarterioles sampled from the same part of the lung specimen were selected and images were taken. After the samples were cleaned and dried by ultrasonication, gold plating was added using an ion coating machine (JMB – 3500 va). The samples were observed using a scanning electron microscope (Hitachi S – 3400 n) to select different regions, and the microvascular diameters were measured using Image J software.
2.4 Measurement of blood physiological indexes
A hematology analyzer (GRT-6008) was used to measure the blood parameters of 12 pigs.
2.5 Quantitative real-time PCR (qRT-PCR) analysis of the expression of hypoxia-related genes
Total RNA was extracted using the total RNA extraction kit from Biotech (TianGen, China), and the HiScript® II 1st strand cDNA synthesis kit (Vazyme, China) was used for the reverse transcription of cDNA. Subsequently, ChamQ™ Universal SYBR® qPCR Master Mix (Vazyme) was used for real-time fluorescence quantitative analysis. Primer sequences for the HIF-1α, EPAS1, EPO, VEGF, eNOS, EGLN1 and GAPDH (reference) genes were used for qRT-PCR (Table S1). The transcript abundance was calculated using the following formula: 2(ΔCt Test – ΔCt Control). The data were analyzed using the 2−Ct method.
2.6 Statistical analysis
The differences in the pulmonary artery morphology of the lungs in Tibetan and Landrace pigs were analyzed and compared. The SPSS 19.0 software was used for the data analyses. Intergroup comparisons were performed by one-way ANOVA, and Duncan's method was used for multiple comparisons. The diameters, blood physiological indexes and gene expression levels in the pulmonary arteries of Tibetan pigs and Landrace pigs were analyzed, and P<0.05 indicated a significant difference.
3.1 Differences in lung morphologies
In this study, the analysis of the pulmonary morphology revealed some differences in size and type between Tibetan (TGN and TJC) and Landrace pigs (LYD and LJC) (Fig. 1, Table 1). Although the lungs of Tibetan pigs were markedly smaller than those of Landrace pigs, the weight of adult Tibetan pigs was also lower than that of Landrace pigs; thus, we used the ratio of lung tissue to individual weight to represent the relative weight of the lungs. Surprisingly, the ratio obtained for Tibetan pigs was significantly higher than that found for Landrace pigs (P<0.05). The density of Tibetan pigs was significantly higher than that of Landrace pigs (P<0.05); in contrast, the ratio of the major axis to the minor axis found for Tibetan pigs was lower than that obtained for Landrace pigs. These findings indicated that the lungs of Tibetan pigs were heavier and wider than those of Landrace pigs.
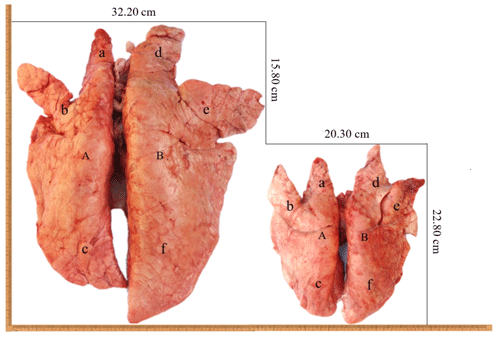
Figure 1The lungs of Tibetan pigs (right) and Landrace pigs (left). A: left lung; B: right lung; a: left anterior lobe; b: left middle lobe; c: left rear lobe; d: right anterior lobe; e: right middle lobe; f: right rear lobe.
Table 1Pulmonary morphological indicators.

Note: because the Tibetan and Landrace pigs exhibited major differences in body weight, we compared the ratio of the lung weight to the body weight. Values with the same superscripts in the same row did not differ significantly (P>0.05), and values with different superscripts in the same row differed significantly (P<0.05).
3.2 Differences in the anatomical architecture and diameter of the pulmonary artery
The anatomical structure of the pulmonary artery was clearly observed using the cast specimens (Fig. 2). The number, relative position and direction of the aorta and its branches were clearly discernible. An analysis of the pulmonary artery specimens from Tibetan and Landrace pigs indicated that the distribution pattern of the arteries was shaped like a tree branch. The pulmonary artery is divided into three parts, including the ascending branch and the downward branch, which are shaped like an arch. Several flexible arterioles are divided at different angles; the first branch exhibits the smallest angle, and the arterioles, which are shaped like a tree branch, show the largest angle. The comparison of the left lungs of Tibetan and Landrace pigs revealed that the numbers of first pulmonary arteries in these pigs were 23–27 and 28–32, respectively. In addition, Tibetan pigs had more and denser arterioles than Landrace pigs, and the pulmonary artery branch of Tibetan pigs presented a larger angle at the same site than that of Landrace pigs.
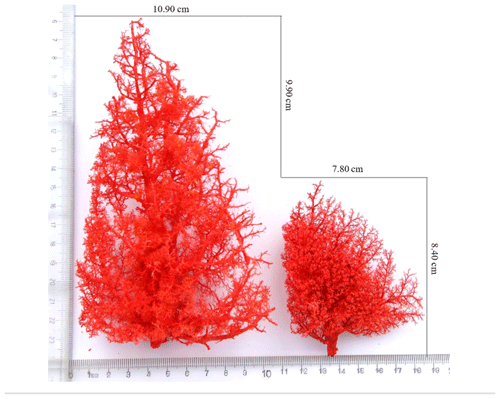
Figure 2Morphological features of the pulmonary artery. Tibetan pig cast specimens of the pulmonary artery are on the left, and Landrace pig cast specimens of the pulmonary artery are on the right.
We compared the relative diameters of the pulmonary arteries of the four groups and branches based on the weight of Tibetan and Landrace pigs at different altitudes. The results showed similar arterial distributions and variations in diameter between the groups, and the diameter decreased with increases in the branch level. The major / minor axis ratio of Tibetan pigs was lower than that of Landrace pigs. The average diameter of the main pulmonary artery and branches of Tibetan pigs was significantly larger than that of Landrace pigs (P<0.05), and the diameters of the high-altitude pigs were larger than those at low altitudes (P>0.05).
Table 2Diameters of the left pulmonary artery of pigs.
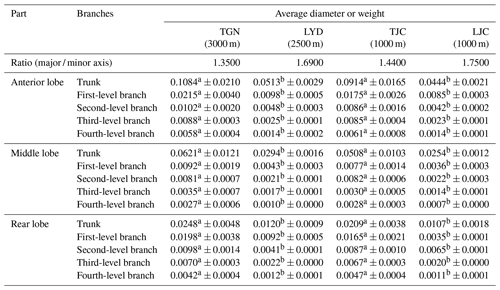
Notes: values with the same superscripts in the same row did not significantly differ (P>0.05), and values with different superscripts in the same row differed significantly (P<0.05).
3.3 Characteristics of arterioles observed by scanning electron microscopy
A scanning electron microscopy analysis revealed that the pulmonary artery surface of Tibetan and Landrace pigs was rough and had a “dendritic” shape (Fig. 3a–d). A three-dimensional analysis revealed that each trunk produced second- to fourth-grade branches at different angles. Each branch had an increasing number of branches from the aorta to the arterioles. In the arterial process, the branch twisted and turned at a certain angle, and a smaller diameter was associated with increased folding. The arterioles with diameters of less than 0.42 mm had branches at a 90∘ angle with obtuse branches at the end (Fig. 3e). The left pulmonary arterioles with a 72.64–123.89 µm diameter on the surface were distributed in endothelial cell indentations, which were distributed unevenly and largely in LYD, evenly and deeply in TGN, and poorly and shallowly in TJC and were not found in LJC. Most endothelial cell indentations were shaped like a boat or footprint, and the length and width were approximately 6.55–9.88 and 3.22–4.51 µm, respectively (Fig. 3f–i). However, the surface dents of the precapillary arterioles with diameters of approximately 18.23–22.23 µm were smooth in all four sets of pigs. We observed a deeper annular constriction on the surface of micro-vessels in the high-altitude pigs than in the low-altitude pigs.
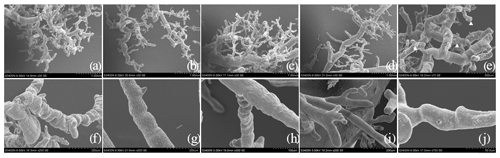
Figure 3Distribution and superficial features of the arterioles in the left lung. (a) The arteriole distribution of the LYD group (×30). (b) The arteriole distribution of the TGN group (×20). (c) The arteriole distribution of the TJC group (×30). (d) The arteriole distribution of the LJC group (×30). (e) Microvascular terminal, with a triangle mark (×70). (f) Imprints of the endothelial nuclei of the LYD group (×200). (g) Imprints of the endothelial nuclei of the TGN group (×200). (h) Imprints of the endothelial nuclei of the TJC group (×300). (i) Imprints of the endothelial nuclei of the LJC group (×200). (j) Precapillary arterioles (×700).
3.4 Determination of blood biochemical indexes
The measurement of various blood biochemical indicators related to the oxygen concentration in the pigs showed that the RBC number of LYD was significantly larger than that of TJC. The HGB, corpuscular hemoglobin concentration (MCHC) and Hb* values of the high-altitude pigs were significantly higher than those of the low-altitude pigs. The hematocrit (HCT), mean corpuscular volume (MCV), mean corpuscular hemoglobin (MCH), red cell volume distribution width (RDW-CV) and red blood cell distribution width standard deviation (RDW-SD) values of the TGN group were significantly larger than those of the other groups.
3.5 Expression of genes correlated with hypoxia
The qRT-PCR analysis confirmed that the expression levels of HIF-1α, EPAS1, EPO, VEGF, eNOS and EGLN1 differed between the different breeds (Fig. 4). The expression levels of HIF-1α, EPAS1, EPO and VEGF in the lungs of TGN and LYD were significantly higher than those in the lungs of TJC and LJC (P<0.05), and the highest levels of these genes were found in the TGN group. The expression of VEGF was highest in the TGN group and lowest in the LJC group, and the diameter of the pulmonary artery exhibited a similar trend. A comprehensive analysis showed that the expression levels of EPO and RBC were higher in the high-altitude pigs than in the low-altitude pigs. Notably, the expression of eNOS in the TJC and LJC groups was significantly higher than that in the TGN and LYD groups, and the expression of EGLN1 in TJC was significantly higher than that in TGN (P<0.05) and negatively correlated with the HGB.
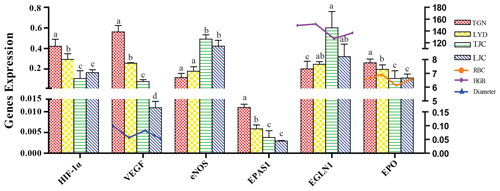
Figure 4Expression levels of HIF-1α, EPAS1, EPO, VEGF, eNOS and EGLN1 in the lungs of the four types of pigs and the relationship between VEGF, EPO and EGLN1 and diameter, RBCs and HGB. Note: the different letters above the bars indicate that the expression levels differed significantly (P<0.05, mean ± SE), and the same letters indicate that the levels did not differ significantly (P>0.05, mean ± SE) in different breeds.
4.1 Structure and function of the pulmonary artery of pigs
To adapt to the hypoxic environment of the plateau, the lungs of plateau animals gradually increased in size during evolution, which is beneficial for enhancing the blood capillary and respiratory area. These findings were also found in our studies (Bai et al., 2012). The presence of multiple branches and a large respiratory region increase the area of air exchange in the lungs, which is necessary for increased lung ventilation and a higher gas exchange rate in animals living in a high-altitude hypoxic environment. The diameter of the pulmonary arteries that supply blood to the lungs of Tibetan sheep is larger than that of Small-tailed Han sheep (Jia et al., 2018). In addition, the contents of capillaries in the alveolar septum of plateau animals, including yak and Tibetan sheep, were richer than those of plain animals. Developed blood vessels can supply sufficient blood to the lungs to increase the number of alveoli and provide rich nutrients for lung growth and development, which is conducive to accelerating the transport of blood oxygen (Qi et al., 2019; Jia et al., 2018; Zhang et al., 2015a). The developed lungs and arteries of Tibetan pigs can reduce the vascular resistance and increase the blood flow, which is conducive to improving the flow of blood and oxygen to the pulmonary artery system to alleviate the insufficient supply of oxygen at high altitudes.
Vascular endothelial cells are widely distributed in various tissues and are the only cells in contact with blood (Yang et al., 2019). Vascular smooth muscle cells, which are generally shaped like “tapers”, play an important role in regulating the blood flow rate and maintaining vascular tension (Wang et al., 2015). Some studies have found that the surfaces of the cerebral and heart arteries of yak and Tibetan sheep resemble “bark”, and the indentation on the surface is shaped like a taper (He et al., 2007). Vascular endothelial cells with a lamellar and lamelliform appearance are conducive to the absorption of liquid from plasma and its transporting to tissues. Large finger-like features are used to buffer rapid blood flow in large vessels and facilitate material exchange (Sturtzel et al., 2017; Li et al., 2014). Due to their exposure to low temperature, plateau animals often appear sluggish and show constricted peripheral blood vessels. We found that the lungs of Tibetan pigs present a greater arteriolar distribution, larger diameter, more capillaries and more dents on the surface than those of Landrace pigs. These findings indicated that Tibetan pigs exhibit an increased blood supply in the lungs, which is conducive to overcoming the obstruction of the respiratory system caused by the high-altitude and low-oxygen environment.
4.2 Blood biochemical indexes of pigs
This study showed that the RBC and HGB concentrations of pigs at high altitudes (TGN and LYD) were significantly higher than those at low altitudes (TJC and LJC). The increase in RBCs induced by a higher altitude is conducive to overcoming the high-altitude and low-oxygen environment, which is consistent with the results from an immigration test of Tibetan pigs and is conducive to improving the body's tolerance to hypoxic environments (Zhang et al., 2015b).
Compared with lowland cattle, domestic yaks have developed physiological features adapted to high-altitude hypoxic conditions, such as a thinner alveolar septum and a thinner blood–air barrier (Zhou et al., 2015). The native highlander Tibetans also acquired similar physiological features, such as an enhanced hypoxic and hypercapnic ventilatory responsiveness, an improved lung diffusion capacity, minimal hypoxic pulmonary hypertension, high levels of exhaled nitric oxide (NO), and low levels of HGB (Qi et al., 2015) . In addition, both domestic yaks and Tibetans maintain a normal blood HGB level compared with that of lowland cattle and Han populations, respectively. These findings suggest that highland Tibetans and domestic yak likely have similar strategies (red cell overproduction) to protect themselves from high-altitude polycythemia (Zhang et al., 2015; Beall et al., 1998). Earlier studies have proposed that high HGB concentrations are conducive to long-term living in a hypoxic environment (Beall et al., 2002). These physiological features might facilitate efficient blood flow for oxygen delivery under hypobaric hypoxia. Therefore, HGB is considered the key factor for individual fitness in hypoxic environments. The blood indexes of Tibetan pigs living in plateau (3000 m) and flatland habitats (1000 m) were measured, and these measurements revealed that those at high altitude presented significant reductions in the hematocrit level, average erythrocyte volume and erythrocyte distribution width and decreases in the leucocyte and average erythrocyte HGB levels compared with those at low altitude (Xu et al., 2019). HGB, a complex allosteric iron-containing protein in vertebrate RBCs, is formed from the combination of heme and globin, which is a key indicator of respiratory movement and could transport oxygen and carbon dioxide and maintain the acid–base balance in blood (Wajcman et al., 2002).
High HGB concentrations might be an adaptive mechanism linked to hemodynamic mechanisms that induce blood thickening, affect the transport of oxygen in blood and eventually cause chronic altitude sickness (CMS) (Beall et al., 1998, 2002). Therefore, HGB was once considered the key factor of fitness in a hypoxic environment. The RBC, HGB and hematocrit levels might be responsible for the compensatory increase in RBCs in hypoxic environments through the proliferation and synthesis of HGB, which improves the oxygen-carrying ability of pigs and allows their migration to a plateau environment from plains through their adaption to high-altitude hypoxic environments (Xu et al., 2019; Wajcman et al., 2002; Zhang et al., 2019). A higher level of HGB is associated with a higher oxygen affinity and a higher tolerance to hypoxia. A series of indicators, such as the measured reductions in the hematocrit level and erythrocyte volume, maintained a low blood viscosity, which is conducive to the smooth circulation of the blood and a reduction in the heart burden (Yang et al., 2017; Tang et al., 2019). All of these factors could provide an important hematological basis for the mechanism underlying the adaptation of Tibetan pigs to high altitude.
4.3 Differential expression of hypoxia-related genes in the lung and their associations with the morphology and physiology of pigs
Tibetan pigs can survive on the plateau environment due to their adaptation to hypoxic conditions, and the normal functioning of the lung is mainly regulated by angiogenesis, cellular energy metabolism and the expression of hypoxia-related genes. These processes are generally associated with HIF, which is a heterodimer consisting of the HIF-1α and HIF-1β subunits and a member of the basic helix–loop–helix PER–ARNT–SIM (bHLH-PAS) protein family (Chertok et al., 2018).
The HIF-1α subunit degrades under normoxic conditions but is stable under hypoxic conditions, whereas the HIF-1β subunit is constitutively expressed. HIF-1α and EPAS1 can regulate hundreds of genes, such as the positive regulators VEGF, EPO and eNOS and the negative regulator EGLN1 (Yu et al., 2018; Bhattarai et al., 2018; Yu et al., 2020). The EGLN1 gene is an important oxygen-sensing gene [4], and a decreased hydroxylation activity of EGLN1 could lead to gradual increases in the expression of HIF-1α and HIF-2α. EPAS1, which is the only gene located within a 70 kb region on chromosome 3 in Tibetan pigs, encodes the HIF-2α protein and is responsible for an increased regulation of many hypoxia-induced genes, including EPO, VEGF and eNOS (Yu et al., 2018; Xu et al., 2018). Hypoxia is the main cause of EPO secretion. As a traditional indicator of erythropoiesis and HGB, EPO is the first hypoxic fitness indicator proposed, and the pig EPO gene is located on chromosome 3 and consists of four introns and five exons. Elevated EPO amplifies the proliferation and differentiation of RBC precursors and increases HGB levels (Deji et al., 2015; Auzmendi et al., 2020). VEGF, as a target gene of HIF-1α, can promote endothelial cell proliferation and neo-vascularization and increase vascular permeability (Yu et al., 2018). HIF-1α is positively correlated with VEGF and increases with increases in hypoxia at high altitudes (Dzhalilova et al., 2018). In our studies, the high expression of HIF-1α, EPAS1, EPO and the normal expression of VEGF and eNOS in the high-altitude pigs were consistent with the large artery diameter and the high number of vascular branches found in these pigs. Increases in the hematocrit level and the blood viscosity can improve the oxygen-carrying capacity of blood as well as the symptoms of hypoxia. NO, which is expressed in multiple cell types as a vasodilator, and eNOS produced by NO can regulate the vasoconstriction and dilatation of blood vessels, which could induce the relaxation of smooth muscles and the dilation of arteries to regulate the blood pressure and blood flow distribution (Ghosh et al., 2018). EPAS1 can enhance the expression of eNOS and eventually lead to an increase in the NO levels, and reduced oxygen levels and increased blood flow facilitate the supply of oxygen to the lungs (Yang et al., 2019). Some studies have shown that long-term exposure to a hypoxic environment inhibits eNOS activity, reduces eNOS expression in mouse lung tissues, decreases the production and release of NO, weakens the vasodilatory effects, promotes the proliferation of pulmonary vascular endothelial cells and smooth muscle cells, enhances pulmonary vascular remodeling, and increases the pulmonary artery pressure (Janaszak-Jasiecka et al., 2018). The activity and expression of eNOS were inhibited in the lung tissues of Landrace pigs after migration from the lowland to the plateau environment, and the difference in eNOS expression between Tibetan and Landrace pigs was obvious and consistent with the results of our study (Zhang et al., 2013). Recent studies have also indicated that EGLN1 exhibits a significant negative correlation with the HGB levels. The high expression of EGLN1 in lung tissues of Tibetan and Landrace pigs at high altitudes is negatively correlated with the HGB concentration (Zhang et al., 2013). Therefore, the expression of genes related to oxygen adaptation, vasodilation and an increase in the number of capillaries, RBC count and HGB concentration might be the biological regulatory mechanism underlying the adaptation of Tibetan pigs to the high-altitude and low-oxygen environment of the plateau.
This study demonstrates that the lungs of Tibetan pigs are heavier and wider than those of Landrace pigs and that Tibetan pigs have a higher number of and denser arteries and arterioles than Landrace pigs. The analysis of the lung structure revealed that the high-altitude Tibetan pigs had more developed lungs and abundant arteries to ensure their respiration in a low-oxygen environment. In addition, the HIF-1 signaling pathway might be one of the major factors that influence the RBC count, HGB level and number of arterioles, which might be regulated through the environment. The findings also show that the regulation of the lung function of Tibetan pigs in a hypoxic environment is manifested at various levels to ensure breathing under extreme environmental conditions.
The data are available from the corresponding author upon request.
The supplement related to this article is available online at: https://doi.org/10.5194/aab-64-283-2021-supplement.
YY conceived and designed the experiments. YY, CG and TY performed the experiments. YY, YS, YC, XW and QY analyzed the data. YY, CL, BW and SZ contributed reagents, materials or analysis tools. YY and SZ wrote the paper.
The authors declare that they have no conflict of interest.
This research has been supported by the National Natural Science Foundation of China (grant nos. 31760644 and 32060730).
This paper was edited by Steffen Maak and reviewed by two anonymous referees.
Ai, H., Yang, B., Li, J., Xie, X., Chen, H., and Ren, J.: Population history and genomic signatures for high-altitude adaptation in Tibetan pigs, BMC Genomics., 15, 834, https://doi.org/10.1186/1471-2164-15-834, 2014.
Auzmendi, J., Puchulu, M. B., Garcia, R. J., Balaszczuk, A. M., Lazarowski, A., and Merelli, A.: EPO and EPO-receptor system as potential actionable mechanism to protection of brain and heart in refractory epilepsy and SUDEP, Curr. Pharm. Des., 26, 1356–1364, https://doi.org/10.2174/1381612826666200219095548, 2020.
Bai, M., Shang, P., Liu, J., Dan, Z., De, J., Wang, H., and Qiang, B.: Preliminary study on pulmonary tissue and hypoxia adaptation to plateau for Tibetan pigs, Hubei Agr. Sci., 51, 2776–2779, https://doi.org/10.14088/j.cnki.issn0439-8114.2012.13.064, 2012 (in Chinese with English Abstract).
Beall, C. M., Brittenham, G. M., Strohl, K. P., Blangero, J., Williams-Blangero, S., Goldstein, M. C., Decker, M. J., Vargas, E., Villena, M., Soria, R., Alarcon, A. M., and Gonzales, C.: Hemoglobin concentration of high-altitude Tibetans and Bolivian Aymara, Am. J. Phys. Anthropol., 106, 385–400, https://doi.org/10.1002/(SICI)1096-8644(199807)106:3<385::AID-AJPA10>3.0.CO;2-X, 1998.
Beall, C. M., Decker, M. J., Brittenham, G. M., Kushner, I., Gebremedhin, A., and Strohl, K. P.: An ethiopian pattern of human adaptation to high-altitude hypoxia, P. Natl. Acad. Sci. USA, 99, 17215–17218, https://doi.org/10.1073/pnas.252649199, 2002.
Bhattarai, D., Xu, X., and Lee, K.: Hypoxia-inducible factor-1 (HIF-1) inhibitors from the last decade (2007 to 2016): A “structure-activity relationship” perspective, Med. Res. Rev., 38, 1404–1442, https://doi.org/10.1002/med.21477, 2018.
Bi, J., Hu, B., Wang, J., Liu, X., Zheng, J., Wang, D., and Xiao, W.: Beluga whale pVHL enhances HIF-2α activity via inducing HIF-2α proteasomal degradation under hypoxia, Oncotarget., 8, 42272–42287, https://doi.org/10.18632/oncotarget.15038, 2017.
Cheng, Y., Lin, C., Chen, J. Y., Li, C. H., Liu, Y. T., and Chen, B. C.: Induction of connective tissue growth factor expression by hypoxia in human lung fibroblasts via the MEKK1/MEK1/ERK1/GLI-1/GLI-2 and AP-1 pathways, PLoS ONE, 11, e0160593, https://doi.org/10.1371/journal.pone.0160593, 2016.
Chertok, V. M., Nevzorova, V. A., and Zakharchuk, N. V.: Comparative study of HIF-1α- and HIF-2α-immunopositive neurons and capillaries in rat cortex under conditions of tissue hypoxia, Bull. Exp. Biol. Med., 165, 516–520, https://doi.org/10.1007/s10517-018-4207-6, 2018.
Deji, B. Z., Shang, P., Danzeng, W. J., Zhang, H., and Qiangba, Y. Z.: Expression and hypoxia adaptation analysis of the EPO gene in different tissues of plateau Tibetan pigs, Genet. Mol. Res., 14, 1700–1706, https://doi.org/10.4238/2015.March.6.16, 2015.
Dzhalilova, D. S., Diatroptov, M. E., Tsvetkov, I. S., Makarova, O. V., and Kuznetsov, S. L.: Expression of Hif-1α, Nf-κb, and Vegf genes in the liver and blood serum levels of HIF-1α, erythropoietin, VEGF, TGF-β, 8-isoprostane, and corticosterone in wistar Rats with High and Low Resistance to Hypoxia, Bull. Exp. Biol. Med., 165, 781–785, https://doi.org/10.1007/s10517-018-4264-x, 2018.
Gan, M., Shen, L., Fan, Y., Guo, Z. X., Bin Liu, Chen, L., Tang, G. Q., Jiang, Y. Z., Li, X. W., Zhang, S. H., Bai, L., and Zhu, L.: High altitude adaptability and meat quality in Tibetan pigs: A reference for local pork processing and genetic improvement, Animals, 9, 1080, https://https://doi.org/10.3390/ani9121080, 2019.
Ghosh, D., Bhargava, K., and Sethy, N. K.: Post-translational modifications of eNOS augment nitric oxide availability and facilitates hypoxia adaptation in Ladakhi women, Nitric Oxide, 78, 103–112, https://doi.org/10.1016/j.niox.2018.06.003, 2018.
He, Y. Y.: Studies on the microvasculature of infant and adult yak's heart. Dissertation, Gansu agricultural university, 2007 (in Chinese with English Abstract).
Ishikawa, H., Xu, L., Sone, K., Kobayashi, T., Wang, G., and Shozu, M.: Hypoxia induces hypoxia-inducible factor 1α and potential HIF-responsive gene expression in uterine leiomyoma, Reprod. Sci., 26, 428–435, https://doi.org/10.1177/1933719118776793, 2019.
Janaszak-Jasiecka, A., Siekierzycka, A., Bartoszewska, S., Serocki, M., Dobrucki, L. W., and Collawn, J. F.: eNOS expression and NO release during hypoxia is inhibited by miR-200b in human endothelial cells, Angiogenesis, 21, 711–724, https://doi.org/10.1007/s10456-018-9620-y, 2018.
Jeong, W., Jung, S., Bazer, F. W., and Kim, J.: Hypoxia-dependent accumulation of hypoxia-inducible factor-1 alpha induces transient cell cycle arrest in porcine trophectoderm cells, Theriogenology, 115, 9–15, https://doi.org/10.1016/j.theriogenology. 2018.04.016, 2018.
Jia, R. L.: Comparison of pulmonary blood vessels in sheep at different altitudes, Shanghai Journal of Animal Husbandry and Veterinary Medicine, 6, 14–15, https://doi.org/10.16656/j.issn.1673-4696.1997.10.039, 1996 (in Chinese with English Abstract).
Kong, X. Y., Gou, X., Ma, T., Leng, J., Mao, H. Y., and Yang, S. L.: Study on blood physiological indicators of adaptation to hypoxia in Tibetan pig, Journal of Yunnan Agricultural University, 29, 297–300, https://doi.org/10.3969/j.issn.1004£390X(n).2014.02.026, 2014 (in Chinese with English Abstract).
Li, P.: Advances in the pathophysiology of vascular endothelial cells, Chinese Journal of Microcirculation, 24, 1–7, 2014.
Ma, Y. F., Han, X. M., Huang, C. P., Zhong, L., Adeola, A. C., Irwin, D. M., Xie, H. B., and Zhang, Y. P.: Population genomics analysis revealed origin and high-altitude adaptation of Tibetan pigs, Sci. Rep., 9, 11463, https://doi.org/10.1038/s41598-019-47711-6, 2019.
Mohamed, A. A.-R., Metwally, M. M., Khalil, S. R., Salem, G. A., and Ali, H. A.: Moringa oleifera extract attenuates the CoCl2 induced hypoxia of rat's brain: Expression pattern of HIF-1α, NF-kB, MAO and EPO, Biomed. Pharmacother., 109, 1688–1697, https://doi.org/10.1016/j.biopha.2018.11.019, 2019.
Moore, L. G., Zamudio, S., Zhuang, J., Sun, S., and Droma, T.: Oxygen transport in Tibetan women during pregnancy at 3,658 m, Am. J. Phys. Anthropol., 114, 42–53, 2001.
Patra, K., Jana, S., Sarkar, A., Mandal, D. P., and Bhattacharjee, S.: The inhibition of hypoxia-induced angiogenesis and metastasis by cinnamaldehyde is mediated by decreasing HIF-1α protein synthesis via PI3K/Akt pathway, BioFactors, Oxford, England, 4, 401–415, https://doi.org/10.1002/biof.1499, 2019.
Qi, X., Zhang, Q., He, Y., Yang, L., Zhang, X., Shi, P., Yang, L. P., Liu, Z. H., Zhang F. H., Liu F. Y., Shiming Liu, Wu T. Y, Cu, C. Y., Ouzhuluobu., Caijuan Bai, C. J., Baimakangzhuo., Han, J. L., Zhao, S. G., Liang, C. N., and Su, B.: The transcriptomic landscape of yaks reveals molecular pathways for high altitude adaptation, Genome Biol. Evol., 11, 72–85, https://doi.org/10.1093/gbe/evy264, 2019.
Sturtzel, C.: Endothelial Cells, Adv. Exp. Med. Biol., 1003, 71–91, https://doi.org/10.1007/978-3-319-57613-8_4, 2017.
Tang, F., Feng, L., Li, R., Wang, W., Liu, H., Yang, Q., and Ge, R. L.: Inhibition of suicidal erythrocyte death by chronic hypoxia, High. Alt. Med. Biol., 20, 112–119, https://doi.org/10.1089/ham.2017.0159, 2019.
Wajcman, H. and Kiger, L.: Hemoglobin, from microorganisms to man: a single structural motif, multiple functions, C. R. Biol., 325, 1159–1174, https://doi.org/10.1016/s1631-0691(02)01537-8, 2002.
Wang, G., Jacquet, L., Karamariti, E., and Xu, Q.: Origin and differentiation of vascular smooth muscle cells, J. Physiol., 593, 3013–3030, https://doi.org/10.1113/JP270033, 2015.
Wang, X. R., Yang, Y. N., and Li, M. N.: Vascular morphology and expression of angiogenic factors in sheep ovaries and their relation with the lambing rate, Reprod. Domest. Anim., 55, 1132–1138, https://doi.org/10.1111/rda.13751, 2020.
Xu, X. H., Bao, Y., Wang, X., Yan, F., Guo, S., Ma, Y., Xu, D., Jin, L., Xu, J., and Wang, J.: Hypoxic-stabilized EPAS1 proteins transactivate DNMT1 and cause promoter hypermethylation and transcription inhibition of EPAS1 in non-small cell lung cancer, FASEB J., https://doi.org/10.1096/fj.201700715, 2018.
Xu, Y. J., Gong, X. L., Duan, M. Q., Wu, L. C., BianBa, Q. D., Guo, X. Y., Qi, Y., Qiangba, Y. Z., and Shang, P.: Determination of blood physiological parameters in Tibetan pigs and Yorkshire pigs at high altitude, J. Plat. Agr., 3, 421–449, https://doi.org/10.19707/j.cnki.jpa.2019.04.011, 2019 (in Chinese with English Abstract).
Yang, Y., Lu, F., Zhuang, L., Yang, S., Kong, Y., Tan, W., Gong, Z., and Zhan, S.: Combined preconditioning with hypoxia and GYKI-52466 protects rats from cerebral ischemic injury by HIF-1α eNOS pathway, Am. J. Transl. Res., 9, 5308–5319, 2017.
Yang, Y., Tian, T., Wang, Y., Li, Z., Xing, K., and Tian, G.: SIRT6 protects vascular endothelial cells from angiotensin II-induced apoptosis and oxidative stress by promoting the activation of Nrf2/ARE signaling, Eur. J. Pharmacol., 859, 172516, https://doi.org/10.1016/j.ejphar.2019.172516, 2019.
Yanyu, H., John, S, M., M, P., Guan, W., and Xiu, L.: Association of age with the expression of hypoxia-inducible factors HIF-1α, HIF-2α, HIF-3α and VEGF in lung and heart of Tibetan sheep, Animals, 9, 673, https://doi.org/10.3390/ani9090673, 2019.
Yu, J., Liang, F., Huang, H., Pirttiniemi, P., and Yu, D.: Effects of loading on chondrocyte hypoxia, HIF-1α and VEGF in the mandibular condylar cartilage of young rats, Orthod. Craniofac. Res., 21, 41–47, https://doi.org/10.1111/ocr.12212, 2018.
Yu, Y., Ma, L., Zhang, H., Sun, W., Zheng, L., Liu, C., and Miao, L.: EPO could be regulated by HIF-1 and promote osteogenesis and accelerate bone repair, Artif. Cells Nanomed. Biotechno.l, 48, 206–217, https://doi.org/10.1080/21691401.2019.1699827, 2020.
Zhang, B., QiangBa, Y. Z., Li, Q. G., Yang, Y. Z., Wang, Z. X., and Ban, D. M.: Endothelial nitric oxide synthase (eNOS) gene expression and hypoxia adaptation analysis in Tibetan pig, Journal of China Agricultural University, 18, 104–108, https://doi.org/10.11841/j.issn.1007-4333.2013.05.16, 2013 (in Chinese with English Abstract).
Zhang, R., Yu, X., Shen, Y., Yang, C., Liu, F., Ye, S., Du, X., Ma, L., Cao, H., Wang, Z., and Li, C.: Correlation between RBC changes and coagulation parameters in high altitude population, Hematology, 24, 325–330, https://doi.org/10.1080/16078454.2019.1568658, 2019.
Zhang, S., Li, J., Kong, X., and Yan, D.: Research progress of animal adaptation to hypoxia, Jo. Anhui Agr. Sci., 43, 148–153, https://doi.org/10.13989/j.cnki.0517-6611.2015.12.054, 2015a (in Chinese with English Abstract).
Zhang, Y., Xu, X., and Yu, H.: A preliminary study on the physiological and biochemical indexes of Tibetan pig blood in Shi Hezi, Gansu animal husbandry and veterinary, 45, 51–52, https://doi.org/10.15979/j.cnki.cn62-1064/s.2015.05.020, 2015b (in Chinese with English Abstract).
Zhao, Y., Lu, X., Cheng, Z., Tian, M., Qiangba, Y., Fu, Q., and Ren, Z.: Comparative proteomic analysis of Tibetan pig spermatozoa at high and low altitudes, BMC Genomics, 20, 569, https://doi.org/10.1186/s12864-019-5873-0, 2019.
Zhou, J., Yu, S., H., J., and Cui, Y.: Comparative structural organization and morphological analyses of intrapulmonary artery of adult yak and cattle, Chinese Journal of Veterinary Medicine, 35, 1840–1862, https://doi.org/10.16303/j.cnki.1005-4545.2015.11.22, 2015 (in Chinese with English Abstract).