the Creative Commons Attribution 4.0 License.
the Creative Commons Attribution 4.0 License.
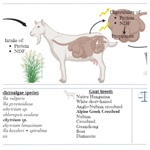
Microalgae supplementation improves goat milk composition and fatty acid profile: a meta-analysis and meta-regression
Soumaya Boukrouh
Ihssane Mnaouer
Poliana Mendes de Souza
Jean-Luc Hornick
Abdelaziz Nilahyane
Bouchra El Amiri
Abdelaziz Hirich
Recently, there has been an emphasis on research on sustainable and environmentally friendly agricultural practices. Microalgae are a promising feed that is rich in essential nutrients, and research has been oriented toward their incorporation into ruminant diets. This study aimed to evaluate the inclusion of microalgae in goat diets using a meta-analysis methodology. The data were acquired from 17 peer-reviewed scientific papers. The raw mean difference between the treatment diets supplemented with microalgae and the control diets was evaluated using the random-effects model. Experimental characteristics such as animal breed, days in milk, experimental duration, microalgae species, inclusion levels, and concentrate were used as covariates in meta-regression and subgrouping analyses. Microalgae supplementation did not affect dry matter intake (DMI, p=0.170) but significantly improved the intake of crude protein (CP, p<0.001) and neutral detergent fiber (NDF, p=0.005). The incorporation of microalgae into the goat diet improved all digestibility parameters (p<0.01), with an improvement in fermentation parameters, including ruminal pH (p=0.010) and propionate (p<0.001). Microalgae inclusion in goat diets increased blood glucose levels (p<0.001) but did not affect blood antioxidant activity (p>0.05). Microalgae supplementation did not affect milk yield (p=0.480) but increased the yield of lactose (p<0.001), protein (p<0.001), and fat (p<0.001). Microalgae inclusion in goat diets improves the fatty acid (FA) profile. The milk of goats had significantly decreased C18:0 (p=0.001) and C18:1 n-9 (p=0.028) and increased C20:5 n-3 (p=0.027), C22:6 n-3 (DHA, p<0.001), polyunsaturated FA (PUFA, p=0.039), and n-3 (p=0.006). Subgroup analysis showed that an inclusion level higher than 30 g per kg DM was advisable to obtain this total improvement. However, even a moderate inclusion level (15–30 g per kg DM) improved the FA profile. The microalgae species Schizochytrium sp. and the Alpine–Greek crossbreed goat breed were covariates that showed interesting results concerning the improvement of DHA and PUFA. In addition, supplementation of goat diets with microalgae could be used as a nutritional approach to enhance milk production and quality.
- Article
(1474 KB) - Full-text XML
- BibTeX
- EndNote
Ruminant milk is one of the most popular beverages in the world. It is a substantial contributor to global food security as an important source of protein, fat, sugar, minerals, and vitamins (Moore et al., 2023). In fact, the world population is envisaged to grow, and inquiries into animal products will increase in parallel (Smith et al., 2022). In Morocco, goat milk production reached 46 227 t in 2018 (FAOSTAT, 2022). Moreover, in rural and dry areas, camel and goat breeding is a fundamental economic activity that contributes to breeder income and cultural heritage (Ait El Alia et al., 2025; Boukrouh et al., 2023a, 2024c).
However, livestock farming increases the pollution from natural resources (Sakadevan and Nguyen, 2017). Intensive livestock farming contributes significantly to air pollution, with the release of greenhouse gases including carbon dioxide methane and nitrous oxide, significantly accelerating climate change (Sakadevan and Nguyen, 2017). Livestock production is associated with nutrient loss, soil degradation, and grassland conservation (Boukrouh et al., 2024a). In this context, research on alternative feedstuffs as a substitute for standard feedstuffs has recently increased (Boukrouh et al., 2023b, c; 2024b; Hirich et al., 2021, 2020), especially in terms of overcoming some environmental problems related to salinity or when the costs of traditionally used feedstuffs are very high.
Microalgae are microscopic photosynthetic organisms capable of transforming sunlight and carbon dioxide (CO2) into valuable biomass. Recently, microalgae cultivation has been developed and used as an unconventional animal feed owing to its environmental and economic advantages (Khan et al., 2018). Algae are highly efficient in converting solar energy, which gives them a rapid growth rate and increases their production compared with traditional crops (Khan et al., 2018). Moreover, microalgae do not rely on external environmental factors. They can substantially valorize water that is unsuitable for humans, thus reducing the pressure on cultivated lands (Holman and Malau-Aduli, 2013).
Microalgae possess abundant essential and healthy unsaturated fatty acids (UFAs), docosahexaenoic acid (DHA), and eicosapentaenoic acid (EPA), which are present in lower amounts in ruminant feeds (Kholif and Olafadehan, 2022). Their composition varies according to the algal growth conditions, species, and genus (Kholif and Olafadehan, 2022). The green freshwater microalgae Chlorella vulgaris, belonging to the family Chlorellaceae and the class Trebouxiophyceae, is a primary source of linoleic (C18:2 n-6) and α-linolenic (C18:3 n-3) beneficial FA (Pantami et al., 2020). The microalgae Japonochytrium sp. is a saprophytic species belonging to the kingdom Thraustochytrid, which produces and accumulates high amounts of DHA in its biomass (Jaseera and Kaladharan, 2020). Schizochytrium sp. microalgae are rich in nutrients, such as sugar and protein, and contain a higher fat content (18.3 % DM–25 % DM) than other microalgae. The FA profile of Schizochytrium sp. is rich in UFA and is composed of palmitic acid (C16:0), docosapentaenoic acid (DPA), and DHA (Zhu et al., 2022). These microalgae UFAs can contribute to improving ruminal fermentation and feed digestibility (Sofyan et al., 2022) and thus to improving the transfer of UFA or conjugated linoleic acid (CLA) to animal products. CLA has anti-atherogenic, anti-carcinogenic, and anti-obesity properties (Vignaud et al., 2023). Microalgae are also a major source of proteins, essential amino acids, and other health-promoting nutrients, making them attractive feed supplements for livestock production (Mavrommatis and Tsiplakou, 2020).
A meta-analysis evaluating microalgae incorporation into goat kid, poultry, and pig diets showed interesting results and performance improvement (Martins et al., 2021; Orzuna-orzuna et al., 2021). Nevertheless, studies on milk production and FA profiles of ruminants incorporating microalgae are still scarce (Martins et al., 2021). Notably, some studies have evaluated the effects of incorporating microalgae into lactating-goat diets on milk production, chemical composition, FA, and fermentation profile. However, the results of these studies are controversial and inconclusive. For example, the incorporation of low doses (5 g) of microalgae into a goat diet increased milk production, chemical composition, FA profile, and fermentation parameters (Kholif et al., 2022). Conversely, the incorporation of low doses in other studies had no significant effect on milk yield and quality (Mavrommatis et al., 2018; Tsiplakou et al., 2018). According to Martins et al. (2021), previous studies have provided valuable insights, but the body of literature remains diverse, encompassing variations in microalgae species, doses of inclusion, animal breeds, and periods of supplementation.
A few recently published review articles have demonstrated the importance of microalgae inclusion in ruminant diets for the improvement of milk production and quality in ruminants (Altomonte et al., 2018; Hernández et al., 2022). However, these studies did not apply meta-analytic methods to assess the effects of microalgae on goats. According to Viechtbauer (2010), meta-analysis is a statistical approach offering unbiased evidence regarding the effectiveness of a treatment by aggregating and quantitatively analyzing data from various previously published studies. The hypothesis of the present meta-analysis asserts that the incorporation of microalgae into lactating-goat diets will ameliorate goat milk production, chemical composition, and FA profile. Consequently, the objective of this study was to evaluate, through a meta-analytic procedure, the impact of dietary supplementation with microalgae on animal performance and milk production and quality.
2.1 Literature search
A systematic literature search was performed using Science Direct, Scopus, PubMed, and Google Scholar to identify scientific papers that evaluated the effects of microalgae in goat diets. Google Scholar searches were performed by browsing the articles until the results were repeated. The database search was performed thrice. The search approach of the systematic review was based on PICO: population, intervention, comparator, and outcome (Schiavenato and Chu, 2021). The following terms were used for the population: goat or dairy goat. The intervention concerned microalgae in goat diets. The comparator was viewed as an animal receiving the same treatment, with or without microalgae supplementation. The outcome was milk production and quality (milk or lactation). The studies used in the meta-analysis spanned a period of 7 years, starting from the first publication in 2015 to the most recent publication in 2022. The identification, selection, and inclusion of studies adhered to the guidelines outlined in the Preferred Reporting Items for Systematic Reviews and Meta-Analyses (PRISMA) protocol (Al Gharad et al., 2025) (Fig. 1).
The requirements for papers to be included in the meta-analysis were as follows: (i) the papers must be peer-reviewed original research papers published in English that report on, at least, the available lactational performance data in goats, with the data mentioning the standard error (SE) or standard deviation (SD); (ii) the study must have both control and experimental treatments that were fed microalgae; (iii) the experimental diets were free from microalgae protection by fat or any other growth promoters; and (iv) the goats used in the feeding trials were healthy and clinically safe. In total, 17 studies were considered to be suitable for meta-analysis, and a description of the experimental conditions is shown in Table 1.
2.2 Data extraction
Papers meeting the inclusion criteria were rated by publication reference, country, number of animals, animal breed, days in milk, experimental period, microalgae species, inclusion level, and amount of concentrates in the date (g per kg DM). Variables reported in three or more publications were the only variables considered. Data were excluded if duplicates were found in more than one journal. The following parameters were included in the final database: intake of dry matter (DMI), organic matter (OMI), crude protein (CPI), and neutral detergent fiber (NDFI); digestibility of DM (DMD), OM (OMD), CP (CPD), NDF (NDFD), ADF (ADFD), and ether extract (EED); fermentation parameters (ruminal pH, NH3, acetate, propionate); blood parameters (total protein, albumin, globulin, glucose); antioxidant activity (superoxide dismutase (SOD), catalase (CAT), glutathione reductase (GR), glutathione transferase (G-Tr), and glutathione peroxidase (G-Px)); milk yield and chemical composition (total solids, ash, fat, protein, solids not-fat, protein, lactose, fat, solids-not fat); and FA profile (stearic (C18:0), oleic (C18:1 n-9), linoleic (C18:2 n-6), conjugated linoleic (C18:2 c9t11), α-linolenic (C18:3 n-3), arachidonic (C20:4 n-3), eicosapentaenoic (C20:5 n-3), docosahexaenoic (C22:6 n-3)) and FA ratios and summaries (saturated FA (SFA), monounsaturated FA (MUFA), polyunsaturated FA (PUFA), n-3, n-6, C14:1 : C14:0, C16:1 : C16:0, and atherogenicity index).
Table 1Summary of the studies included in the meta-analysis.
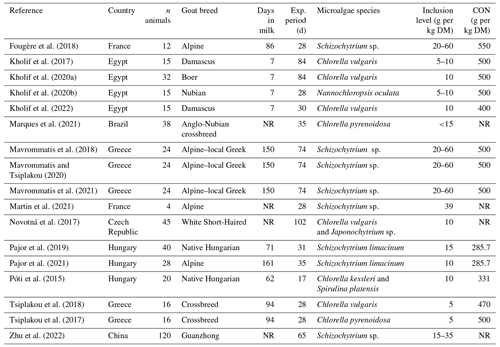
n denotes number, exp. denotes experimental, and CON denotes concentrate in the diet. NR denotes not reported.
The number of repetitions, means, and variances (i.e., SE and SD) were extracted for the control (without microalgae addition) and treatments (with microalgae addition). In studies that reported SE instead of SD, the SE values were converted to SD using the following formula: SD = SE × , where n is the number of goats in each treatment group (Xue et al., 2019). Also, when a study reported the incorporation level in terms of percentage (%) instead of g kg−1, the percentage value was converted to g kg−1 using the following formula: 1 % = 10 g kg−1. In feeding assays with variable treatments, data were only extracted from the microalgae and control groups, irrespective of the other treatments.
2.3 Statistical analysis
Statistical analysis of the collected data was performed using a meta-analysis. Data were analyzed using the “meta” and “metafor” packages of R software (Version 4.4.1). All outcomes in subsequent studies were continuous data. The raw mean difference (RMD) with a 95 % confidence interval (CI) was selected for the calculation and combination of effect measures. The significance of the combined effect sizes was evaluated using the Z test, and treatment had a distinct effect when p≤0.05.
Publication bias was detected using Egger's test (1997), and the bias was considered to be present when p≤0.05. Heterogeneity was quantified using chi-squared (Q) and I2 tests (Riley et al., 2011). In a meta-analysis, I2 indicates the percentage of variance assigned to study heterogeneity. I2 ranges from 0 % to 100 % and is considered to be present when it exceeds 50 % and when p≤0.05 (Langan, 2022).
Meta-regression was used to investigate potential contributors to heterogeneity. The meta-regression criteria for the heterogeneity test and for Egger's test was p≤0.05. Meta-regression analysis were performed using the subsequent covariates: (1) animal breed (native Hungarian, White Short-Haired, Alpine–Greek crossbreed, Nubian, crossbreed, Guanzhong, Boer, Alpine, Damascus, Anglo-Nubian crossbreed), days in milk (<90, 90–180 d), experimental period (<90, >90 d), microalgae species (Chlorella vulgaris, Chlorella pyrenoidosa, Japonochytrium sp., Nannochloropsis oculata, Schizochytrium sp., Schizochytrium limacinum, Chlorella kessleri and Spirulina platensis), microalgae inclusion level (<10, 11–20, 21–30, 31–40, 41–50, 51–60 g per kg DM), and the level of concentrate in the diet (<400, 400–600 g per kg DM).
Subgroup analysis was also used to elucidate heterogeneity when the covariates (from the meta-regression procedure in Table 4) were significant at p≤0.10. The subgroup assessment was conducted for variables reported in at least 10 studies in the meta-analysis. The covariate was compelling with regard to explaining the heterogeneity when the p value for the subgroup differences was less than 0.05 (Table 4).
The database comprises 17 peer-reviewed scientific papers with 29 chosen treatment means to explore the effects of microalgae incorporation into dairy goat diets on their performance and milk quality (Table 1). A total of 953 dairy goats, comprising 1061 comparisons, were used in the meta-analysis.
3.1 Intake, digestibility, and fermentation parameters
The results revealed that there was no evidence of an intervention effect on dry matter intake (DMI, p=0.170) and organic matter intake (OMI, p=0.227). However, a significant difference was found in crude protein intake (CPI, p<0.001) and neutral detergent fiber intake (NDFI, p=0.005) (Table 2). Microalgae supplementation in goat diets significantly improved dry matter digestibility (DMD, p=0.001), organic matter digestibility (OMD, p<0.001), crude protein digestibility (CPD, p=0.001), neutral detergent fiber digestibility (NDFD, p=0.012), acid detergent fiber digestibility (ADFD, p<0.001), and ether extract digestibility (EED, p=0.003). Microalgae supplementation significantly increased the ruminal pH (p=0.010) and propionate (p<0.001). Heterogeneity was discovered in all previous parameters (p<0.05, 61.56<I2<96.22), except in ADFD (p=0.492, I2=0) and propionate (p=0.295, I2=0).
3.2 Blood and antioxidant parameters
Glucose levels were significantly increased by the incorporation of microalgae into goat diets (p<0.001), whereas no significant effect was observed for total protein (p=0.093), albumin (p=0.524), and globulin (p=0.253) (Table 3). Antioxidant activity was not affected by microalgae incorporation for SOD (p=0.073), CAT (p=0.536), GR (p=0.363), G-Tr (p=0.059), or G-Px (p=0.436). Concerning blood parameters, heterogeneity was reported only for albumin (p<0.001, I2=95.13), SOD (p<0.001, I2=92.51), GR (p<0.001, I2=95.04), and G-Tr (p<0001, I2=91.38).
3.3 Milk composition and fatty acid profile
The effects of microalgae incorporation on chemical composition and milk FA profile are reported in Table 3. The addition of microalgae into goat diets did not affect milk yield (p=0.480) but increased the yield of lactose (p<0.001), protein (p<0.001), fat (p<0.001), and solid non-fats (p<0.001). Heterogeneity was observed for all milk chemical composition parameters (p<0.001, 62.62<I2<100), except for protein yield (p=0.786, I2=0) and lactose (p=0.885, I2=0).
Feeding microalgae to lactating goats affected individual FA and significantly decreased C18:0 (p=0.001) and C18:1 n-9 (p=0.028). This incorporation into goat diets significantly increased C20:5 n-3 (p=0.027) and C22:6 n-3 (p<0.001). This also improved the summaries of FAs, including polyunsaturated FA (PUFA, p=0.039) and n-3 (p=0.006). Microalgae incorporation significantly decreased the C14:1 : C14:0 desaturase index (p= 0.030) and the atherogenicity index (AI; p=0.016). Heterogeneity was observed for all FAs (p<0.001, 88.50<I2<100).
3.4 Meta-regression analysis
As shown in Tables 2–4, the presence of publication bias from Egger's test was not evident for all parameters (p>0.05). Meta-regression analysis was used to investigate the primary contributors to variation among the response variables. The results are shown in Figs. 2, 3, and 4. Among the covariates, animal breed, microalgae species, and inclusion level were the major variables influencing digestibility and fermentation parameters, blood antioxidant activity, milk yield, chemical composition, and FA profile. For response variables in which the covariates accounted for less than 50 % of the heterogeneity, such as DMI (adjusted R2=47.35), DMD (adjusted R2=48.71), OMD (adjusted R2=34.96), total solids (adjusted R2=19.56), protein (adjusted R2=34.75), and glutathione reductase (adjusted R2=7.06), unidentified factors not captured in our meta-analysis might have influenced the impact of microalgae supplementation in goat diets. Thus, these parameters were not included in the subgroup analysis.
Table 3Effects of microalgal supplementation on milk yield, chemical composition, and fatty acid profile of dairy goats.
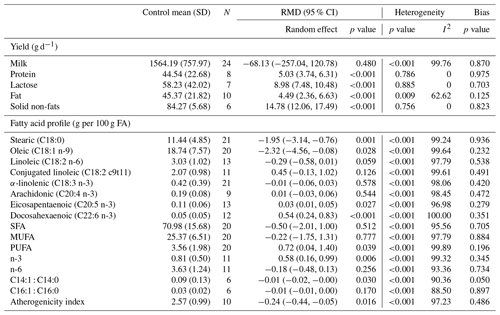
N denotes number of comparisons, SD denotes standard deviation, CI denotes confidence interval, SFA denotes saturated fatty acid MUFA denotes monounsaturated FA, and PUFA denotes polyunsaturated FA.
Table 4Meta-regression of covariate effect on raw mean differences (RMDs) between microalgae and control treatments.
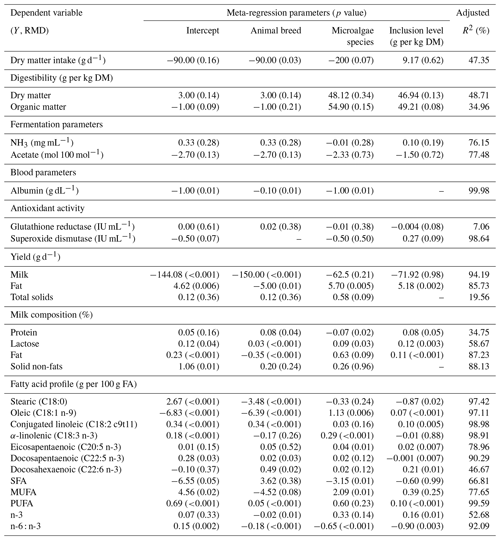
Regarding the effects of the animal breed covariate, microalgae incorporation into goat diets reduced milk yield for the White Short-Haired breed (p<0.001), whereas no change was observed in the other animal breeds. Total solids increased when microalgae were introduced into Boer (p<0.001) and Damascus (p=0.005) diets and did not change for the other breeds. Milk fat significantly increased in the native Hungarian breed (p=0.019) and decreased in Alpine–Greek crossbreeds (p=0.013). Milk from Damascus goats showed significant decreases in C18:2 c9t11 (p=0.006) and increased C18:0 (p=0.023), C18:1 n-9 (p<0.001), C18:3 n-3 (p=0.010), C22:5 n-3 (p<0.001), and C22:6 n-3 (p=0.009) when supplemented with microalgae. When microalgae was incorporated into the Alpine–Greek crossbreed diet, there was a significant decrease in C18:0 (p=0.003) and C18:1 n-9 (p<0.001) and a significant increase in C18:2 c9t11 (p<0.001), C18:3 n-3 (p=0.010), C22:5 n-3 (p<0.001), C22:6 n-3 (p=0.009), PUFA (p<0.001), and n-3 (p<0.001). For the Boer breed, microalgae incorporation increased C18:0 (p=0.031) and C18:1 n-9 (p<0.001) and decreased C18:2 c9t11 (p<0.001). Nubian goats showed significant increases in C18:0 (p=0.007), C18:1 n-9 (p<0.001), C18:3 n-3 (p=0.016), and MUFA (p=0.020) and a significant decrease in C18:2 c9t11 (p<0.001). Native Hungarians showed increased C18:1 n-9 (p<0.001) and C18:3 n-3 (p=0.003).
Microalgae species also differ in terms of their effects on goat milk and performance. Incorporation of Schizochytrium sp. into goat diets significantly decreased milk C18:0 (p=0.002), C18:1 n-9 (p<0.001), C18:3 n-3 (p<0.001), and MUFA (p=0.035) and significantly increased C20:5 n-3 (p=0.012), C22:6 n-3 (p=0.014), and PUFA (p<0.001). The incorporation of Japonochytrium sp. decreased milk yield (p=0.026) and C18:3 n-3 content (p<0.001). When Chlorella vulgaris was incorporated into goat diets, an increase in TS (p=0.018) and a decrease in C18:2 c9t11 (p=0.010), C18:3 n-3 (p<0.001), and C22:5 n-3 (p=0.008) were observed. The incorporation of Schizochytrium limacum increased SFA (p=0.003) and decreased C18:1 n-9 (p=0.005), C18:3 n-3 (p<0.001), C20:5 n-3 (p=0.013), C22:5 n-3 (p=0.006), and MUFA (p=0.010). When Nannochloropsis oculata was incorporated into goat diets, a decrease in C18:3 n-3 (p<0.001) and C22:5 n-3 (p=0.015) was observed.
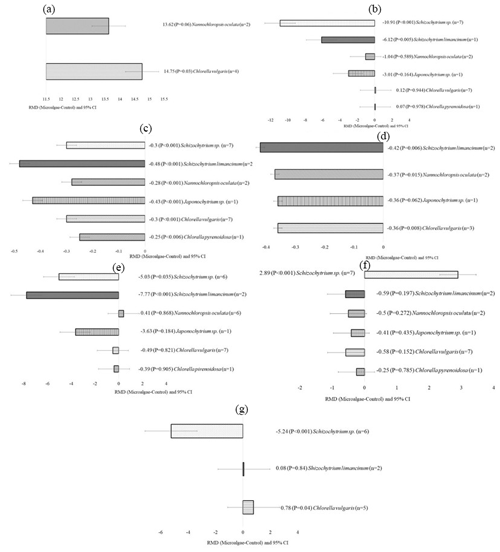
Figure 2Subgroup analysis (subgroup refers to microalgae species) of microalgae incorporated into dairy goats. RMD denotes raw mean difference between treatment and control diets; n denotes the number of comparisons. (a) Fat (g per 100 g FA), (b) C18:1 n-9 (g per 100 g FA), (c) C18:3 n-3 (g per 100 g FA), (d) C20:5 n-3 (g per 100 g FA), (e) monounsaturated fatty acids (g per 100 g FA), (f) polyunsaturated fatty acids (g per 100 g FA), (g) n-6 : n-3.
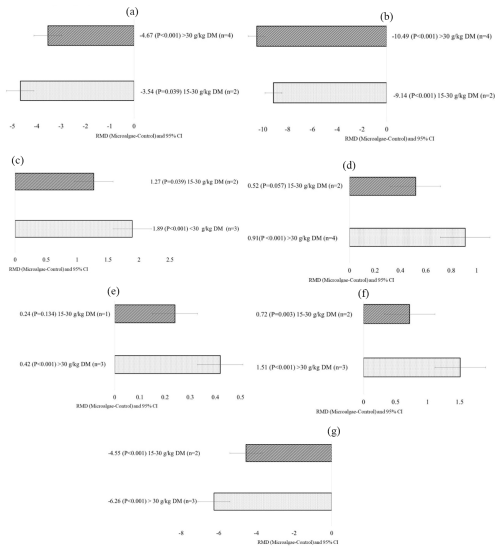
Figure 3Subgroup analysis (subgroup refers to inclusion level) of microalgae incorporated into dairy goats. RMD denotes raw mean difference between treatment and control diets; n denotes number of comparisons. (a) C18:0 (g per 100 g FA), (b) C18:1 n-9 (g per 100 g FA), (c) C18:2 c9t11 (g per 100 g FA), (d) C22:6 n-3 (g per 100 g FA), (e) C22:5 n-3 (g per 100 g FA), (f) n-3 (g per 100 g FA), (g) n-6 : n-3.
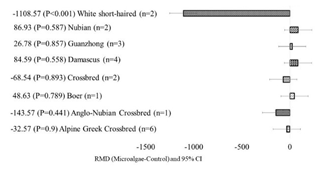
Figure 4Subgroup analysis (subgroup refers to goat breed) of microalgae incorporated into dairy goats. RMD denotes raw mean difference between treatment and control diets; n denotes number of comparisons. Milk yield in g d−1.
The quality and production of goat milk were also affected by the level of microalgae incorporation. Higher levels of microalgae incorporation (>30 g per kg DM) significantly increased C18:2 c9t11 (p<0.001), C20:5 n-3 (p=0.001), C22:5 n-3 (p<0.001), C22:6 n-3 (p<0.001), PUFA (p<0.001), and n-3 (p<0.001) and significantly decreased C18:0 (p<0.001) and C18:1 n-9 (p<0.001). Moderate incorporation of 15–30 g per kg DM microalgae increased C18:2 c9t11 (p=0.039), PUFA (p<0.001), and n-3 (p=0.003) and decreased C18:0 (p=0.039) and C18:1 n-9 (p<0.001).
4.1 Overview of studies used in the analysis
The findings from this meta-analysis indicate that the majority of studies will be conducted from 2015 to 2022. This trend might be associated with the global initiative urging researchers to explore alternative natural feed supplements to enhance livestock productivity. This research spanned eight countries, representing four continents, which shows that research on microalgae as animal feed is emerging worldwide.
4.2 Microalgae overall effect
Ruminants have been fed microalgae as an alternative, low-cost, and highly digestible feed (Niccolai et al., 2019). The first expected barrier to feeding large quantities of microalgae to ruminants is their palatability problems due to vegetable, grass, and cucumber flavors (Van Durme et al., 2013). In a subsequent study, DM intake was not affected by microalgae incorporation into goat diets, while a meta-analysis of small ruminants receiving microalgae showed an improved intake (Orzuna-Orzuna et al., 2023). As expected, microalgae improved the digestibility of DM, OM, CP, EE, and fibers (NDF and ADF). The improved nutrient digestibility indicates heightened rumen microflora activity following microalgal supplementation. Microalgae possess approximately 67 % UFA in terms of their overall total FA content (Maltsev and Maltseva, 2021), and supplementation with UFA may have decreased the number of protozoa known to engulf bacterial cells. Boeckaert et al. (2007) observed that microalgae with high levels of UFA reduced the populations of certain ciliates, including Isotricha intestinalis, Isotricha prostoma, and Epidinium caudatum. The decrease in the number of protozoa is linked to reduced predation of the bacterial population and improved digestibility (Kholif and Olafadehan, 2021).
For fermentation parameters, microalgae supplementation in goat diets did not affect ruminal acetate, which might indicate that the levels of UFA present in the microalgae in the current experiment were adequate to improve the performance of goats without affecting ruminal cellulolytic bacterial activity. Moreover, microalgal supplementation of goats increased ruminal pH and propionate levels (Fig. 2). Propionate is considered to be the primary gluconeogenic volatile FA used for lactose and glucose biosynthesis (Wang et al., 2023), which explains the improvement in serum glucose concentrations. Approximately 73 % of the glucose produced in the livers of ruminants originates from ruminal propionic acid (Oh et al., 2015). The incorporation of microalgae into ruminant diets aims to improve milk yield and quality. Contrarily to our hypothesis, the meta-analysis conducted herein outlined that microalgae changed milk quality and composition but not yield. Although microalgal diets are rich in polyunsaturated fatty acids, milk fat depression (MFD) is avoided, and milk fat is improved.
Regarding the phytochemical composition of microalgae, several studies have reported the presence of molecules such as polysaccharides, sterols, vitamins, and pigments (e.g., carotenoids), which have antioxidant roles (Coulombier et al., 2021). However, no significant effect was observed in this meta-analysis. Unexpectedly, in a study conducted on the same species aiming to enrich goat products with PUFA via microalgae supplementation, high dietary levels of Schizochytrium sp. (40 and 60 g d−) increased nicotinamide adenine dinucleotide phosphate (NADPH) oxidase activity in blood plasma, resulting in superoxide anion formation and pro-oxidation, whereas no effect was observed with lower supplementation (20 g). The following observation emphasizes the importance of using the correct dosage, which is highlighted by subgroup analysis. In a study conducted on sheep, Christodoulou et al. (2023) reported that Spirulina dietary supplementation significantly increased the antioxidant activity of animals through higher activities of superoxide dismutase, glutathione peroxidase, and catalase.
Microalgae are sources of PUFA that are reported to inhibit rumen biohydrogenation and, as a result, increase CLA and C18:1 trans-11 substrates (Boukrouh et al., 2023a). These latter FAs can also increase through the activity of Δ9 desaturase in the mammary gland. As milk C14:0 is synthesized de novo in the udder and because milk C14:1 originates exclusively from C14:0 desaturation at this site (Lock and Garnsworthy, 2003), the Δ9 : C14 ratio is considered to be the marker for Δ9 desaturase activity, which was decreased in the following study. In a study comparing fish oil and microalgae effects on goat milk quality, no significant effect was observed for goats receiving microalgae, while there was an improved effect for goats receiving fish oil (Beyzi and Dallı, 2023).
4.3 Microalgae species
The response to microalgae incorporation differed according to microalgae species. In the case of Chlorella vulgaris, despite its higher concentration in C18:2 n-6, isomerization of C18:2 n-6 to CLA was inhibited when it was incorporated into goat diets, which explains the decrease in CLA in milk (Tsiplakou et al., 2018). The reason for the increased C22:6 n-3 and PUFA with the incorporation of Schizochytrium sp. into goat diets compared to other microalgae species is probably the higher concentration in these FAs and the inhibition of ruminal biohydrogenation (Półbrat et al., 2021). A previous study has reported that the DHA content of Schizochytrium sp. accounts for 35 % of the total FA content (Adarme-Vega et al., 2012). Moreover, the higher concentration of PUFA compared to Chlorella vulgaris could be the reason for the reduced fat content. These PUFA are known to modulate rumen biohydrogenation, leading to the accumulation of biohydrogenation intermediates such as trans-10, cis-12 conjugated linoleic acid, which are potential inhibitors of de novo milk fat synthesis, leading to MFD (Baumgard et al., 2001). The improvement in C18:3 n-3 and C22:5 n-3 content when Nannochloropsis oculata was fed to goats could be due to its higher content of n-3 FA, DHA, and EPA (3.2 % and 21.5 % fat, respectively) (Durmic et al., 2014). In addition, C18:3 n-3 is the precursor of n-3 FAs such as EPA and DHA, which are essential for many human metabolic processes and serve as preventive measures against coronary heart disease (Bhat et al., 2023).
4.4 Supplementation level
The level of microalgae incorporation into goat diets affects animal production and performance. Some studies have suggested that supplementation with low doses of microalgae decreases DNA damage and enhances the blood oxidative status in goats and sheep (Vignaud et al., 2023). We expected improvements in general health and increased production. Moderate incorporation of microalgae (15–30 g per kg DM) improved only CLA, PUFA, and n-3 compared to higher incorporation (>30 g per kg DM). However, even moderate incorporation can be beneficial to health. An improvement in n-3 FA can lower human blood triglycerides, reduce the risk factors for coronary heart disease, and minimize the likelihood of thrombosis that leads to heart attack (Yagi et al., 2017).
Stearic acid (C18:0) is the ultimate product of the ruminal biohydrogenation process (Dewanckele et al., 2020). The decrease in stearic acid in the milk of goats receiving both medium (15–30 g per kg DM) and high levels of microalgae (>30 g per kg DM) suggests that the biohydrogenation process is sensitive even to moderate doses of microalgae incorporation. The lower biohydrogenation of PUFA reduced the availability of C18:0 in the mammary gland, which led to the termination of de novo synthesis of C18:1 n-9 through Δ9 desaturase activity (Dewanckele et al., 2020). CLA was raised in the milk of goats that received medium- and high-microalgae diets as a result of the partial inhibition of dietary PUFA biohydrogenation.
4.5 Animal breeds
The impact of animal breed on the goat reaction to microalgae supplementation may be due to differences in rumen microbial communities among goat breeds (Shi et al., 2008). According to Morand-Fehr et al. (2007), variations in milk yield among different goat breeds across various countries can be attributed to differences in genetic and environmental factors, as well as the type of management systems implemented in these regions. Shamay et al. (2000) suggested that, under stressful environmental conditions, the milk composition of goats remains unaffected if they are well-adapted to their environment.
In a study comparing the milk composition of different goat breeds, the lowest fat content was registered for the Alpine breed compared to Damascus and Boer breeds (Ferro et al., 2017), which could explain the decreased milk fat when microalgae were supplemented in the diets of Alpine–Greek crossbreeds as the potential of crossbreeds could not exceed that of Damascus and Boer (Ferro et al., 2017). When selecting for higher milk yield, the concentration of milk fat could decrease due to dilution, which could also be the reason for the decreased milk fat for Alpine–Greek crossbreeds (Goetsch et al., 2011). However, Alpine–Greek crossbreeds showed increased milk C18:3 n-3, CLA, DPA, DHA, PUFA, and n-3 when animals were fed microalgae compared to other breeds, which could be due to the concentration of efforts to enhance the milk quality of the hybrid. It is noteworthy that certain breeds (e.g., White Short-Haired, Boer) and microalgae species have been represented by few studies (often just one), which underscores the necessity for additional research to thoroughly investigate these interactions. Although the meta-analysis employed a random-effects model to account for heterogeneity, the validity of the conclusions drawn for underrepresented subgroups remains limited, and the variability emphasizes the need for more studies to comprehensively explore these interactions. Further research should address these gaps by including a broader range of breeds, experimental conditions, and microalgae species to strengthen the evidence base and to provide more conclusive insights into the role of microalgae supplementation in goat nutrition.
4.6 Investigation of heterogeneity and publication bias
Heterogeneity in meta-analysis is a test used to detect the difference between studies, and high heterogeneity values (I2>50 %) observed in our analysis reflect the expected variability between studies, implying different incorporation methods in goats. The heterogeneity was also highlighted by the higher SD for some variables and probably arises from variability in factors such as diet composition, microalgae species, experimental design and duration, and animal breed. To account for this, we employed a random-effects model (Langan, 2022), which provides a robust estimate in the presence of heterogeneity, assuming that the true effects vary across studies.
Another important parameter in meta-analyses is publication bias, which is generally caused by the orientation of reviewers and journal editors to favor the publication of studies showing significantly positive results rather than those reporting negative findings (Nosek et al., 2022). In the present meta-analysis, despite the high heterogeneity, the absence of significant publication bias suggests that the variability is probably due to natural differences between studies rather than biased reporting of the results, which strengthens the reliability of the overall conclusions. Meta-regression and subgroup analyses were used as a result of high heterogeneity to identify sources of variation and to better contextualize the findings. The employed moderators, such as microalgae species, inclusion levels, and animal breeds, provided a deeper understanding of the conditions where microalgae supplementation is most beneficial.
Research on microalgae as an alternative feed for goats has recently emerged. This meta-analysis provides fundamental information concerning the potential effects of microalgal supplementation on goat milk production and quality. The results showed that microalgae supplementation in goat diets did not affect dry matter intake or milk production, but the FA profile of milk was improved. Our findings also suggest that supplementation with >30 g per kg DM is necessary to improve the milk FA profile and to produce healthier goat milk. The best FA profile was obtained with the use of Schizochytrium sp. and when microalgae were incorporated into the Alpine–Greek crossbreed. The results revealed the presence of heterogeneity but the absence of publication bias, and meta-regression analysis illustrated that covariates explained some of the sources of variation. The aforementioned results may serve as a valuable reference for animal nutritionists and policymakers, helping them make evidence-based decisions concerning the utilization of microalgae feed supplements to enhance goat performance.
The software code presented in this study is available upon request from the corresponding author.
The datasets presented in this study are available upon request from the corresponding author.
SB and JLH designed the study protocol. Data were collected and analyzed by SB and JLH. The manuscript was prepared and edited by SB, JLH, IM, PSM, BE, AN, and AH. All authors contributed to the article and approved the submitted version.
The contact author has declared that none of the authors has any competing interests.
Ethical consent was not required for this study as this is a review paper with no original research data.
Publisher's note: Copernicus Publications remains neutral with regard to jurisdictional claims made in the text, published maps, institutional affiliations, or any other geographical representation in this paper. While Copernicus Publications makes every effort to include appropriate place names, the final responsibility lies with the authors.
The authors express their gratitude to Mohammed VI Polytechnic University (UM6P) for providing financial support for this research.
This research was supported by Mohammed VI Polytechnic University (UM6P).
This paper was edited by Manfred Mielenz and reviewed by Necmettin Unal and Gaurav Kr Deshwal.
Adarme-Vega, T. C., Lim, D. K. Y., Timmins, M., Vernen, F., Li, Y., and Schenk, P. M.: Microalgal biofactories: a promising approach towards sustainable omega-3 fatty acid production, Microb. Cell Fact., 11, 96, https://doi.org/10.1186/1475-2859-11-96, 2012.
Ait El Alia, O., Zine-Eddine, Y., Chaji, S., Boukrouh, S., Boutoial, K., and Faye, B.: Global Camel Milk Industry: A Comprehensive Overview of Production, Consumption Trends, Market Evolution, and Value Chain Efficiency, Small Ruminant Res., 107441, 2025.
Al Rharad, A., El Aayadi, S., Avril, C., Souradjou, A., Sow, F., Camara, Y., Hornick, J.-L., and Boukrouh, S.: Meta-Analysis of Dietary Tannins in Small Ruminant Diets: Effects on Growth Performance, Serum Metabolites, Antioxidant Status, Ruminal Fermentation, Meat Quality, and Fatty Acid Profile, Animals, 15, 596, https://doi.org/10.3390/ani15040596, 2025.
Altomonte, I., Salari, F., Licitra, R., and Martini, M.: Use of microalgae in ruminant nutrition and implications on milk quality–A review, Livest. Sci., 214, 25–35, https://doi.org/10.1016/j.livsci.2018.05.006, 2018.
Baumgard, L. H., Sangster, J. K., and Bauman, D. E.: Milk Fat Synthesis in Dairy Cows Is Progressively Reduced by Increasing Supplemental Amounts of trans-10, cis-12 Conjugated Linoleic Acid (CLA), J. Nutr., 131, 1764–1769, https://doi.org/10.1093/jn/131.6.1764, 2001.
Beyzi, S. B. and Dallı, C. Ç.: Changes in the rumen and milk fatty acid profile and milk composition in response to fish and microalgae oils supplementation to diet alone or combination in dairy goats, Trop. Anim. Health Prod., 55, 407, https://doi.org/10.1007/s11250-023-03824-9, 2023.
Bhat, S., Sarkar, S., Zaffar, D., Dandona, P., and Kalyani, R. R.: Omega-3 Fatty Acids in Cardiovascular Disease and Diabetes: A Review of Recent Evidence, Curr. Cardiol. Rep., 25, 51–65, 2023.
Boeckaert, C., Vlaeminck, B., Mestdagh, J., and Fievez, V.: In vitro examination of DHA-edible micro algae: 1. Effect on rumen lipolysis and biohydrogenation of linoleic and linolenic acids, Anim. Feed Sci. Technol., 136, 63–79, https://doi.org/10.1016/j.anifeedsci.2006.08.015, 2007.
Boukrouh, S., Noutfia, A., Moula, N., Avril, C., Hornick, J.-L., Chentouf, M., and Cabaraux, J.-F.: Effects of sulla flexuosa hay as alternative feed resource on goat’s milk production and quality, Animals, 13, 709, https://doi.org/10.3390/ani13040709, 2023a.
Boukrouh, S., Noutfia, A., Moula, N., Avril, C., Louvieaux, J., Hornick, J. L., Chentouf, M., and Cabaraux, J. F.: Ecological, morpho-agronomical, and nutritional characteristics of Sulla flexuosa (L.) Medik. ecotypes, Sci. Rep., 13, 13300, https://doi.org/10.1038/s41598-023-40148-y, 2023b.
Boukrouh, S., Noutfia, A., Moula, N., Avril, C., Louvieaux, J., Hornick, J. L., Cabaraux, J. F., and Chentouf, M.: Ecological, morpho-agronomical, and bromatological assessment of sorghum ecotypes in Northern Morocco, Sci. Rep., 13, 15548, https://doi.org/10.1038/s41598-023-41565-9, 2023c.
Boukrouh, S., Bouazzaoui, Y., El Aich, A., Mahyou, H., Chikhaoui, M., Ait Lafkih, M., N’Dorma, O., and Alados, C. L.: Estimation of standing crop biomass in rangelands of the Middle Atlas mountains using remote sensing data, Afr. J. Range Forage Sci., 41, 1–16, https://doi.org/10.2989/10220119.2024.2360991, 2024a.
Boukrouh, S., Noutfia, A., Moula, N., Avril, C., Louvieaux, J., Hornick, J.-L., Chentouf, M., and Cabaraux, J.-F.: Characterisation of bitter vetch (Vicia ervilia (L.) Willd) ecotypes: An ancient and promising legume, Exp. Agric., 60, e19, 1–18, https://doi.org/10.1017/S0014479724000139, 2024b.
Boukrouh, S., Noutfia, A., Moula, N., Avril, C., Louvieaux, J., Hornick, J.-L., Cabaraux, J.-F., and Chentouf, M.: Growth performance, carcass characteristics, fatty acid profile, and meat quality of male goat kids supplemented by alternative feed resources: bitter vetch and sorghum grains, Arch. Anim. Breed., 67, 481–492, https://doi.org/10.5194/aab-67-481-2024, 2024c.
Christodoulou, C., Kotsampasi, B., Dotas, V., Simoni, M., Righi, F., and Tsiplakou, E.: The effect of Spirulina supplementation in ewes’ oxidative status and milk quality, Anim. Feed Sci. Technol., 295, 115544, https://doi.org/10.1016/j.anifeedsci.2022.115544, 2023.
Coulombier, N., Jauffrais, T., and Lebouvier, N.: Antioxidant Compounds from Microalgae: A Review, Mar. Drugs, 19, 549, https://doi.org/10.3390/md19100549, 2021.
Dewanckele, L., Toral, P. G., Vlaeminck, B., and Fievez, V.: Invited review: Role of rumen biohydrogenation intermediates and rumen microbes in diet-induced milk fat depression: An update, J. Dairy Sci., 103, 7655–7681, https://doi.org/10.3168/jds.2019-17662, 2020.
Durmic, Z., Moate, P. J., Eckard, R., Revell, D. K., Williams, R., and Vercoe, P. E.: In vitro screening of selected feed additives, plant essential oils and plant extracts for rumen methane mitigation, J. Sci. Food Agric., 94, 1191–1196, https://doi.org/10.1002/jsfa.6396, 2014.
Egger, M., Smith, G. D., Schneider, M., and Minder, C.: Bias in meta-analysis detected by a simple, graphical test, BMJ, 315, 629–634, 1997.
FAOSTAT: Food and Agiculture Organization of the United Nations, https://www.fao.org/faostat/en/#data/QCL (last access: 20 March 2024), 2022.
Ferro, M. M., Tedeschi, L. O., and Atzori, A. S.: The comparison of the lactation and milk yield and composition of selected breeds of sheep and goats, Transl. Anim. Sci., 1, 498–506, https://doi.org/10.2527/tas2017.0056, 2017.
Goetsch, A. L., Zeng, S. S., and Gipson, T. A.: Factors affecting goat milk production and quality, Small Ruminant Res., 101, 55–63, 2011.
Hernández, H., Nunes, M. C., Prista, C., and Raymundo, A.: Innovative and Healthier Dairy Products through the Addition of Microalgae: A Review, Foods, 11, 755, https://doi.org/10.3390/foods11050755, 2022.
Hirich, A., Choukr-Allah, R., and Ragab, R.: Emerging research in alternative crops, Springer, https://doi.org/10.1007/978-3-319-90472-6, 2020.
Hirich, A., Choukr-Allah, R., Ezzaiar, R., Shabbir, S. A., and Lyamani, A.: Introduction of alternative crops as a solution to groundwater and soil salinization in the Laayoune area, South Morocco, EuroMediterr. J. Environ. Integr., 6, 52, 2021.
Holman, B. W. B. and Malau-Aduli, A. E. O.: Spirulina as a livestock supplement and animal feed, J. Anim. Physiol. Anim. Nutr. (Berl.), 97, 615–623, 2013.
Jaseera, K. V. and Kaladharan, P.: Overview of systematics, morphology, biodiversity and potential utilisation of Thraustochytrids, Journal of the Marine Biological Association of India, 62, 13–21, 2020.
Khan, M. I., Shin, J. H., and Kim, J. D.: The promising future of microalgae: current status, challenges, and optimization of a sustainable and renewable industry for biofuels, feed, and other products, Microb. Cell Fact., 17, 36, https://doi.org/10.1186/s12934-018-0879-x, 2018.
Kholif, A. E. and Olafadehan, O. A.: Microalgae in Ruminant Nutrition: a Review of the Chemical Composition and Nutritive Value, Ann. Anim. Sci., 21, 789–806, https://doi.org/10.2478/aoas-2020-0117, 2021.
Kholif, A. E. and Olafadehan, O. A.: Dietary strategies to enrich milk with healthy fatty acids – A review, Ann. Anim. Sci., 22, 523–536, 2022.
Kholif, A. E., Morsy, T. A., Matloup, O. H., Anele, U. Y., Mohamed, A. G., and El-Sayed, A. B.: Dietary Chlorella vulgaris microalgae improves feed utilization, milk production and concentrations of conjugated linoleic acids in the milk of Damascus goats, J. Agric. Sci., 155, 508–518, https://doi.org/10.1017/S0021859616000824, 2017.
Kholif, A. E., Hamdon, H. A., Kassab, A. Y., Farahat, E. S. A., Azzaz, H. H., Matloup, O. H., Mohamed, A. G., and Anele, U. Y.: Chlorella vulgaris microalgae and/or copper supplementation enhanced feed intake, nutrient digestibility, ruminal fermentation, blood metabolites and lactational performance of Boer goat, J. Anim. Physiol. Anim. Nutr. (Berl), 104, 1595–1605, https://doi.org/10.1111/jpn.13378, 2020a.
Kholif, A. E., Gouda, G. A., and Hamdon, H. A.: Performance and milk composition of Nubian goats as affected by increasing level of Nannochloropsis oculata microalgae, Animals, 10, 2453, https://doi.org/10.3390/ani10122453, 2020b.
Kholif, A. E., Gouda, G. A., Abu Elella, A. A., and Patra, A. K.: Replacing the Concentrate Feed Mixture with Moringa oleifera Leaves Silage and Chlorella vulgaris Microalgae Mixture in Diets of Damascus Goats: Lactation Performance, Nutrient Utilization, and Ruminal Fermentation, Animals, 12, 1589, https://doi.org/10.3390/ani12121589, 2022.
Langan, D.: Assessing heterogeneity in random-effects meta-analysis, Meta-research: methods and protocols, 67–89, 2022.
Lock, A. L. and Garnsworthy, P. C.: Seasonal variation in milk conjugated linoleic acid and Δ9-desaturase activity in dairy cows, Livest. Prod. Sci., 79, 47–59, https://doi.org/10.1016/S0301-6226(02)00118-5, 2003.
Maltsev, Y. and Maltseva, K.: Fatty acids of microalgae: diversity and applications, Rev. Environ. Sci. Biotechnol., 20, 515–547, https://doi.org/10.1007/s11157-021-09571-3, 2021.
Marques, I. T. O., Vasconcelos, F. R., Alves, J. P. M., Montenegro, A. R., Fernandes, C. C. L., Oliveira, F. B. B., Silva, C. P., Nagano, C. S., Figueiredo, F. C., and Beserra, F. J.: Proteome of milk fat globule membrane and mammary gland tissue in goat fed different lipid supplementation, Small Ruminant Res., 199, 106378, https://doi.org/10.1016/j.smallrumres.2021.106378, 2021.
Martin, C., Coppa, M., Fougère, H., Bougouin, A., Baumont, R., Eugène, M., and Bernard, L.: Diets supplemented with corn oil and wheat starch, marine algae, or hydrogenated palm oil modulate methane emissions similarly in dairy goats and cows, but not feeding behavior, Anim. Feed Sci. Technol., 272, 114783, https://doi.org/10.1016/j.anifeedsci.2020.114783, 2021.
Martins, C. F., Ribeiro, D. M., Costa, M., Coelho, D., Alfaia, C. M., Lordelo, M., Almeida, A. M., Freire, J. P. B., and Prates, J. A. M.: Using Microalgae as a Sustainable Feed Resource to Enhance Quality and Nutritional Value of Pork and Poultry Meat, Foods, 10, 2933, https://doi.org/10.3390/foods10122933, 2021.
Mavrommatis, A. and Tsiplakou, E.: The impact of the dietary supplementation level with Schizochytrium sp. on milk chemical composition and fatty acid profile, of both blood plasma and milk of goats, Small Ruminant Res., 193, 106252, https://doi.org/10.1016/j.smallrumres.2020.106252, 2020.
Mavrommatis, A., Chronopoulou, E. G., Sotirakoglou, K., Labrou, N. E., Zervas, G., and Tsiplakou, E.: The impact of the dietary supplementation level with schizochytrium sp, on the oxidative capacity of both goats' organism and milk, Livest. Sci., 218, 37–43, https://doi.org/10.1016/j.livsci.2018.10.014, 2018.
Mavrommatis, A., Skliros, D., Simoni, M., Righi, F., Flemetakis, E., and Tsiplakou, E.: Alterations in the rumen particle-associated microbiota of goats in response to dietary supplementation levels of Schizochytrium spp., Sustainability, 13, 607, https://doi.org/10.3390/su13020607, 2021.
Moore, S. S., Costa, A., Pozza, M., Vamerali, T., Niero, G., Censi, S., and De Marchi, M.: How animal milk and plant-based alternatives diverge in terms of fatty acid, amino acid, and mineral composition, NPJ Sci. Food, 7, 50, https://doi.org/10.1038/s41538-023-00227-w, 2023.
Morand-Fehr, P., Fedele, V., Decandia, M., and Le Frileux, Y.: Influence of farming and feeding systems on composition and quality of goat and sheep milk, Small Ruminant Res., 68, 20–34, https://doi.org/10.1016/j.smallrumres.2006.09.019, 2007.
Niccolai, A., Chini Zittelli, G., Rodolfi, L., Biondi, N., and Tredici, M. R.: Microalgae of interest as food source: Biochemical composition and digestibility, Algal. Res., 42, 101617, https://doi.org/10.1016/j.algal.2019.101617, 2019.
Nosek, B. A., Hardwicke, T. E., Moshontz, H., Allard, A., Corker, K. S., Dreber, A., Fidler, F., Hilgard, J., Kline Struhl, M., and Nuijten, M. B.: Replicability, robustness, and reproducibility in psychological science, Annu. Rev. Psychol., 73, 719–748, 2022.
Novotná, K., Fantová, M., Nohejlová, L., Stádník, L., Ducháček, J., and Borková, M.: Effect of Chlorella vulgaris and Japonochytrium sp. microalgae supplementation on composition and fatty acid profile of goat milk, Acta Universitatis Agriculturae et Silviculturae Mendelianae Brunensis, 65, https://doi.org/10.11118/actaun201765051585, 2017.
Oh, Y. K., Eun, J. S., Lee, S. C., Chu, G. M., Lee, S. S., and Moon, Y. H.: Responses of blood glucose, insulin, glucagon, and fatty acids to intraruminal infusion of propionate in Hanwoo, Asian-Australas. J. Anim. Sci., 28, 200, 2015.
Orzuna-orzuna, J. F., Dorantes-iturbide, G., Lara-bueno, A., and Miranda-Romero, L. A.: Growth Performance, Meat Quality and Antioxidant Status of Sheep Supplemented with Tannins: A Meta-Analysis, Animals, 11, 3184, https://doi.org/10.3390/ani11113184, 2021.
Orzuna-Orzuna, J. F., Chay-Canul, A. J., and Lara-Bueno, A.: Performance, milk fatty acid profile and oxidative status of lactating small ruminants supplemented with microalgae: A meta-analysis, Small Ruminant Res., 226, 107031, https://doi.org/10.1016/j.smallrumres.2023.107031, 2023.
Pajor, F., Egerszegi, I., Steiber, O., Bodnár, Á., and Póti, P.: Effect of marine algae supplementation on the fatty acid profile of milk of dairy goats kept indoor and on pasture, https://doi.org/10.22358/jafs/109955/2019, 2019.
Pajor, F., Egerszegi, I., Szűcs, Á., Poti, P., and Bodnár, Á.: Effect of marine algae supplementation on somatic cell count, prevalence of udder pathogens, and fatty acid profile of dairy goats’ milk, Animals, 11, 1097, https://doi.org/10.3390/ani11041097, 2021.
Pantami, H. A., Ahamad Bustamam, M. S., Nakakuni, M., Shaari, K., Lee, S. Y., Mohd Faudzi, S. M., and Ismail, I. S.: Comprehensive GCMS and LC-MS/MS Metabolite Profiling of Chlorella vulgaris, Mar. Drugs, 18, 367, https://doi.org/10.3390/md18070367, 2020.
Półbrat, T., Konkol, D., and Korczyński, M.: Optimization of docosahexaenoic acid production by Schizochytrium SP. – A review, Biocatal. Agric. Biotechnol., 35, 102076, https://doi.org/10.1016/j.bcab.2021.102076, 2021.
Póti, P., Pajor, F., Bodnár, Á., Penksza, K., and Köles, P.: Effect of micro-alga supplementation on goat and cow milk fatty acid composition, Chil. J. Agric. Res., 75, 259–263, https://doi.org/10.4067/S0718-58392015000200017, 2015.
Riley, R. D., Higgins, J. P. T., and Deeks, J. J.: Interpretation of random effects meta-analyses, BMJ, 342, d549, https://doi.org/10.1136/bmj.d549, 2011.
Sakadevan, K. and Nguyen, M.-L.: Chapter Four – Livestock Production and Its Impact on Nutrient Pollution and Greenhouse Gas Emissions, in: Advances in Agronomy, edited by: Sparks, D. L., Academic Press, 147–184, https://doi.org/10.1016/bs.agron.2016.10.002, 2017.
Schiavenato, M. and Chu, F.: PICO: What it is and what it is not, Nurse Educ. Pract., 56, 103194, https://doi.org/10.1016/j.nepr.2021.103194, 2021.
Shamay, A., Mabjeesh, S. J., Shapiro, F., and Silanikove, N.: Adrenocorticotrophic hormone and dexamethasone failed to affect milk yield in dairy goats: comparative aspects, Small Ruminant Res., 38, 255–259, https://doi.org/10.1016/S09214488(00)00152-8, 2000.
Shi, P. J., Meng, K., Zhou, Z. G., Wang, Y. R., Diao, Q. Y., and Yao, B.: The host species affects the microbial community in the goat rumen, Lett. Appl. Microbiol., 46, 132–135, 2008.
Smith, N. W., Fletcher, A. J., Hill, J. P., and McNabb, W. C.: Modeling the contribution of milk to global nutrition, Front. Nutr., 8, 716100, https://doi.org/10.3389/fnut.2021.716100, 2022.
Sofyan, A., Irawan, A., Herdian, H., Jasmadi, Harahap, M. A., Sakti, A. A., Suryani, A. E., Novianty, H., Kurniawan, T., Darma, I. N. G., Windarsih, A., and Jayanegara, A.: Effects of various macroalgae species on methane production, rumen fermentation, and ruminant production: A meta-analysis from in vitro and in vivo experiments, Anim. Feed Sci. Technol., 294, 115503, https://doi.org/10.1016/j.anifeedsci.2022.115503, 2022.
Tsiplakou, E., Abdullah, M. A. M., Alexandros, M., Chatzikonstantinou, M., Skliros, D., Sotirakoglou, K., Flemetakis, E., Labrou, N. E., and Zervas, G.: The effect of dietary Chlorella pyrenoidosa inclusion on goats milk chemical composition, fatty acids profile and enzymes activities related to oxidation, Livest. Sci., 197, 106–111, https://doi.org/10.1016/j.livsci.2017.01.014, 2017.
Tsiplakou, E., Abdullah, M. A. M., Mavrommatis, A., Chatzikonstantinou, M., Skliros, D., Sotirakoglou, K., Flemetakis, E., Labrou, N. E., and Zervas, G.: The effect of dietary Chlorella vulgaris inclusion on goat's milk chemical composition, fatty acids profile and enzymes activities related to oxidation, J. Anim. Physiol. Anim. Nutr. (Berl.), 102, 142–151, https://doi.org/10.1111/jpn.12671, 2018.
Van Durme, J., Goiris, K., De Winne, A., De Cooman, L., and Muylaert, K.: Evaluation of the Volatile Composition and Sensory Properties of Five Species of Microalgae, J. Agric. Food Chem., 61, 10881–10890, https://doi.org/10.1021/jf403112k, 2013.
Viechtbauer, W.: Conducting meta-analyses in R with the metafor package, J. Stat. Softw., 36, 1–48, 2010.
Vignaud, J., Loiseau, C., Hérault, J., Mayer, C., Côme, M., Martin, I., and Ulmann, L.: Microalgae Produce Antioxidant Molecules with Potential Preventive Effects on Mitochondrial Functions and Skeletal Muscular Oxidative Stress, Antioxidants, 12, 1050, https://doi.org/10.3390/antiox12051050, 2023.
Wang, K., Xiong, B., and Zhao, X.: Could propionate formation be used to reduce enteric methane emission in ruminants?, Sci. Total Environ., 855, 158867, https://doi.org/10.1016/j.scitotenv.2022.158867, 2023.
Xue, Y., Yang, Y., and Huang, T.: Effects of chronic exercise inter ventions on executive function among children and adolescents: a systematic review with meta-analysis, Br. J. Sports Med., 0, 1–9, https://doi.org/10.1136/bjsports-2018-099825, 2019.
Yagi, S., Fukuda, D., Aihara, K., Akaike, M., Shimabukuro, M., and Sata, M.: n-3 polyunsaturated fatty acids: promising nutrients for preventing cardiovascular disease, J. Atheroscler. Thromb., 24, 999–1010, 2017.
Zhu, H., Wang, X., Zhang, W., Zhang, Y., Zhang, S., Pang, X., Lu, J., and Lv, J.: Dietary Schizochytrium Microalgae Affect the Fatty Acid Profile of Goat Milk: Quantification of Docosahex aenoic Acid (DHA) and Its Distribution at Sn-2 Position, Foods, 11, 2087, https://doi.org/10.3390/foods11142087, 2022.