the Creative Commons Attribution 4.0 License.
the Creative Commons Attribution 4.0 License.
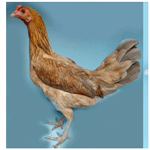
Polymorphism of prolactin (PRL) gene exon 5 and its association with egg production in IPB-D1 chickens
Lailatul Rohmah
Sri Darwati
Niken Ulupi
Isyana Khaerunnisa
Cece Sumantri
The prolactin (PRL) gene regulates the egg production and incubation in laying chickens. Local chickens' reproductive systems will disrupt as a result of the incubation period activity, and they will lay fewer eggs. This study aimed to determine the prolactin gene polymorphism in IPB-D1 hens and its relationship to egg production. The polymorphism of the exon 5 prolactin gene was examined on 112 samples of the IPB-D1 chicken DNA collection from the Division of Animal Genetics and Breeding, Faculty of Animal Sciences, IPB University. By performing the phenol–chloroform method, the genomic DNA was obtained. A polymerase chain reaction (PCR) product with a size of 557 bp was produced as a result of the DNA amplification. Three single-nucleotide sequences were discovered. Three single-nucleotide polymorphisms (SNPs), g.7835A > G, g.7886A > T, and g.8052T > C, were found in exon 5 of the PRL gene. Each mutation was polymorphic and in Hardy–Weinberg equilibrium. The point mutation g.8052T > C significantly impacted the egg production of IPB-D1 chickens, according to the SNP association analysis on egg production, and may serve as a marker to enhance the selection for the features of egg production in IPB-D1 chickens.
- Article
(601 KB) - Full-text XML
- BibTeX
- EndNote
The most crucial economic factors for chickens are reproductive traits, particularly egg production. A chicken's reproductive performance is often reflected in its laying performance. Numerous varieties of chicken have been developed since they were first domesticated for use in food production, entertainment, and other purposes. Commercial breeds or lines are heavily bred for meat or egg production, as opposed to native chicken breeds, which often do not exhibit superior production capability. In previous studies, scientists have developed a new breed to suit Indonesia's demands for animal protein. According to decree no. 693/KPTS/PK.230/M/9/2019, IPB-D1 chickens are the offspring of a cross between Pelung Sentul chickens and native chickens with Cobb parent stock broilers and have received approval from the Indonesian Ministry of Agriculture as a new breed of local superior broiler chickens (Sumantri et al., 2020). Their fast growth and resistance to ND (Newcastle disease) and Salmonella pullorum are the IPB-D1 chickens' advantages (Ulupi et al., 2016; Sumantri and Darwati, 2017; Lukmanudin et al., 2018). IPB-D1 chickens in their seventh generation had an average egg production of 49.22 %, according to study by Habiburahman et al. (2020), which indicates that IPB-D1 chickens have a strong potential to be further developed to generate new lines as female lines with egg production ranging from 50 % to 60 %.
The explorations of single-nucleotide polymorphisms (SNPs) and developments in DNA sequencing technology have initiated a new era of genetic diversity studies in livestock (Gao et al., 2022). SNPs were commonly discovered in the DNA between genes. They can serve as biological markers, guiding researchers to genes linked to disease, qualities and quantities related to production, and also traits related to reproduction. SNPs may have a more direct impact on some features when they occur within a gene or in a regulatory region close to a gene by affecting the gene's function (Shastry, 2009; Goddard, 2016). Finding specific SNPs that are associated with the quantitative trait is a crucial initial step in the process of finding economic and reproductive trait SNPs for breeding.
There have been several attempts to pinpoint the genes that are responsible for variances in chicken egg production. Prolactin is one of the genes associated with the hens' egg production (Smiley, 2019; Susanti, 2015; Zhang, 2012; Wilkanowska, 2014; Bai et al., 2019). A gene candidate called prolactin particularly regulates the variance in egg output by inhibiting egg biosynthesis throughout the incubation periods and is a receptor to control the growth of follicles and the generation of eggs (Mazurowski et al., 2016; Wang et al., 2011; Asiamah et al., 2019). The prolactin gene can be used to assist the selection that will be performed on IPB-D1 hens to generate new strains of female lines.
According to previous studies, the Aves class prolactin hormone has a significant role in the control of physiological processes, such as the growth of gonad cells, the stimulation and maintenance of incubation, egg production, and osmoregulation (Dobolyi et al., 2020). The variety of prolactin genes in Indonesian poultry is presently only known for the native chicken species. According to Lumatauw and Mu'in (2016), the polymorphic prolactin gene information in local chickens is connected to the type of egg production through incubation periods. It has been shown that the SNP at the g.8052T > C position in Chinese local chickens, namely Qingyuan partridge chickens and recessive white chickens, had an influence on the total egg production for the first 300 d of laying eggs. This SNP site is located in exon 5 of the prolactin gene. Additionally, it was discovered that this has a significant impact on the percentage of daily egg production for white Leghorn and Hy-Line Brown laying hens (Erehehuara, 2003; Li et al., 2013). The prolactin gene (PRL) position exon 5 can be utilized as a genetic marker in Indonesian local chicken selection programs, particularly for IPB-D1 chickens, because it has been found to have an association with egg production in chickens. In this study, the variety of prolactin (PRL) genes in IPB-D1 chickens will be identified, and the association between prolactin genetic variation and IPB-D1 chickens' egg production traits will be examined.
2.1 Chicken management and egg collection
IPB-D1 chickens were reared in individual cages under the same conditions in the Breeding and Genetics Field Laboratory of the Faculty of Animal Husbandry at IPB University. During the production period, the chickens were fed with 100 % commercial layer feed, which has a crude protein content of 17 % and a metabolic energy of approximately 2850 kcal kg−1 of feed. Drinking water was provided ad libitum. The eggs were collected daily for 90 d. Blood samples were taken from 112 IPB-D1 chickens in the Field Laboratory of the IPB Faculty of Animal Sciences IPB University. At 24 weeks old, or when the IPB-D1 chicken laid its first egg, blood samples were taken from IPB-D1 chickens. The IPB-D1 chicken was 36 weeks old at the end of the experiment.
The microclimatic conditions during the experiment averaged at 25 ∘C in the morning, 31.4 ∘C at midday, and 28.5 ∘C in the afternoon. The average relative humidity was 89.5 % in the morning, 80.7 % during the day, and 83.1 % in the afternoon. Lighting is provided to the flock from 05:00 to 17:00 LT (local time). Chicken acclimatization was carried out for 3 weeks, and the health of the chickens was monitored every day. All procedures carried out in this study have received approval from the Animal Care and Use Committee (ACUC) of IPB University (ACUC no. 163-2019 IPB). Chicken blood samples were collected from the pectoralis vein in the wing area (as much as 1.0–1.5 mL) using a syringe. The blood is placed into a tube containing the anticoagulant K3-EDTA (ethylenediaminetetraacetic). Until the time for extraction, those blood samples are then kept at 4 ∘C in the refrigerator.
2.2 Genotype of prolactin gene
The National Center for Biotechnology Information's (NCBI) GenBank access code was used to obtain the sequence data for the primer design (AF288765). The author's design for the primer sequence in this investigation used the following primers:
-
forward primer (F), with 5′-TGGAGGAGGCCAAAAAGAGATG-3′, and
-
reverse primer (R), with 5′-TTGCTAAGTACCTGTGGGCTG-3′.
The resultant polymerase chain reaction (PCR) product was 557 bp long and was found at exon 5. A genomic DNA extraction micro-kit (Geneaid Biotech Ltd., Taiwan) was used to extract DNA from fresh blood in accordance with the manufacturer's instructions. A UV transilluminator (Bio-Rad Laboratories, Inc., California, USA) was used to see the PCR products. IPB-D1 chicken sample forward and reverse PCR products were sequenced in Selangor, Malaysia, through first-base services. The chromatographs were analyzed with BLAST, FinchTV, and the MEGA X program (Kumar et al., 2018).
2.3 Data analysis
Calculating the data for genotype frequency, allele frequency, and heterozygosity allowed researchers to examine genetic diversity. The chi-squared test (x2), based on Nei and Kumar's (2000) formula, was used to examine genotype frequency deviations from the Hardy–Weinberg equilibrium, allele frequency, and genotype frequency. The Weir (1996) formula was used to determine the frequency of observed heterozygosity (Ho), and the Nei and Kumar (2000) formula was used to determine the expected heterozygosity (He) based on the allele frequency.
An analysis of the association between the genotype of the prolactin gene and egg production was carried out using the general linear model (GLM) analysis method, namely SAS software. This stage was then followed by Duncan's multiple appeal. The mathematical model used was Yij Gi + εij, according to Mattjik and Sumertajaya (2006), where Yij is the value of egg production, i is the genotype, j is the replication, μ is the common mean, Gi is the genotype effect of i, and Eij is the effect of the experimental error on the i genotype and j replication.
The coefficient of diversity (CD) is a coefficient that shows the degree of accuracy (accuracy or precision) and the reliability of the conclusion of an experiment. The value of the coefficient of diversity can be expressed as the average percent of the general average of the experiment (Harjosuwono et al., 2011).
3.1 Genotype frequency, allele frequency, and heterozygosity value of prolactin gene
The findings from the calculation of the prolactin gene's genotype frequency, allele frequency, and heterozygosity value at various loci are shown in Table 1. According to the findings (Table 1), the three SNPs were polymorphic and had allele frequencies of more than 1 %. The most prevalent genotypes were AG, AA, and TC in the g.7835A > G, g.7886A > T, and g.8052T > C, respectively. Every single SNP discovered is in Hardy–Weinberg equilibrium. The values of observed heterozygosity and predicted heterozygosity (Ho and He, respectively) showed that IPB-D1 chicken diversities were modest. Ho and He had values in all SNPs between 0.09 and 0.54 and 0.08 and 0.49, respectively (Table 2).
Table 1Genotype frequency, allele frequency, heterozygosity and chi-squared test (χ2) prolactin gene SNPs (PRL) in IPB-D1 chickens.
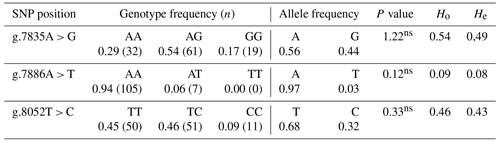
N is the total sample, and n is the number of samples in the genotype. χ2 (0.05; 1) = 3.84, and χ2 (0.05; 2) = 5.99. ∗ is significantly different, and ns is not significantly different (P > 0.05).
Table 2Association of the prolactin SNP gene with egg production in IPB-D1 chickens.
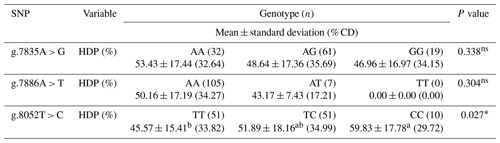
HDP is the hen day production. The numbers on the same line followed by different superscripts show the significant differences at the test level of 5 %, where n is the number of samples, and (% CD) is the percentage coefficient of diversity. ∗ is significantly different, and ns is not significantly different (P > 0.05).
Three diversity indices – locus number (%), mean heterozygosity at Hardy–Weinberg equilibrium, and allele frequency – are used to describe genetic variety in populations (Nei and Kumar, 2000). In a population with genetic variety, the allele frequency measures the relative frequency of each allele. If an allele has a frequency of 0.99 or below, then it is known as polymorphic (Hartl and Clark, 2000). The analysis results (Table 1) indicated that the allele frequency of the point mutation g.7835A > G, g.7886A > T, and 8052 T > C in IPB-D1 chickens was polymorphic because all the allele frequency calculations were less than 0.99. For several genomic prediction processes, allele frequencies are necessary. The utilization of identical allele frequencies is for the base generation, also known as the pedigree founders (Aldridge et al., 2019).
A chi-squared test (χ2) was used in this study to see if the population was in the Hardy–Weinberg equilibrium. If the value of the χ2 count is less than the value in Table 2, then the population is considered to be in equilibrium. When a population is at equilibrium, then it means that there have not been any mutations, migrations, guided marriages, selections, or plenty of samples (Noor, 2010). The findings of the Hardy–Weinberg equilibrium test in Table 2 indicate that all mutation points are either in the Hardy–Weinberg equilibrium or are not statistically different (P > 0.05). According to the results of calculating the heterozygosity values in IPB-D1 hens, the observed heterozygosity value (Ho) was higher than the expected heterozygosity value (He). This shows how diverse the population is that has the mutations at the loci g.7835A > G, g.7886A > T, and 8052T > C. According to Noor (2010), depending on the allele frequency of each locus, heterozygosity is a criterion used to assess the degree of genetic diversity in a population. Due to its stability within a race, the heterozygosity ratio has been suggested as a quality control criterion for SNP data (Guo et al., 2014). The population is diversified, as evidenced by the observed heterozygosity value, which is higher than the expected heterozygosity value.
Table 3Association of the prolactin SNP gene with body weight first on egg in IPB-D1 chickens.
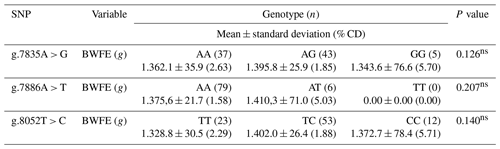
BWFE is the body weight first on egg. The numbers on the same row followed by different letters show significant differences at the test level of 5 %, where n is the number of samples, and (% CD) is the percentage coefficient of diversity. ∗ is significantly different, and ns is not significantly different (P > 0.05).
3.2 Association of SNP with chicken egg production IPB-D1
The association analysis of each SNP of the prolactin gene with the egg production or hen day production (HDP) of IPB-D1 chickens is presented in Table 2. The analysis results in Table 2 show that the mutation g.8052T > C is more associated with the characteristics of IPB-D1 chicken egg production, as compared to other association point mutations. Genotype CC had a higher percentage of hen day production compared to other genotypes on the mutation g.8052T > C. The TT genotype had the lowest average daily egg production, followed by the TC genotype with medium egg production.
The association between DNA polymorphisms and reproductive traits has been found. Therefore, this polymorphism is a potential genetic marker for the marker-assisted selection (MAS) program. The analysis results show that the mutation g.8052T > C is more associated with the characteristics of IPB-D1 chicken egg production, as compared to other mutations (Table 2). This is in line with research conducted by Li et al. (2013), who analyzed two mutation points of T8052C and G8113C in exon 5, using the PCR-LDR (ligase detection reaction) method on local Chinese laying hens, namely Qingyuan partridge chickens and recessive white chickens. The results showed an association of the prolactin (PRL) gene with age for the first laying eggs and the total number of eggs during the first 300 d of laying eggs. According to Erehehuara (2003), the SNPs g.8113G > C and g.8052T > C had a significant impact on the egg production of white Leghorn and Hy-Line Brown chickens. Another study also found the association of prolactin gene exon 5 with Indonesian domestic ducks. Thus, study mentioned that SNP C97T with the CC and CT genotypes exhibited a high egg production and large egg weight (Astuti and Prijono, 2014).
The percentage of daily egg production in IPB-D1 chickens was shown to be significantly affected (P<0.05) by the association point mutation g.8052T > C. The results of this analysis indicated that IPB-D1 chickens with genotype CC had a higher percentage of hen day production compared to other genotypes. IPB-D1 chickens with the TT genotype had the lowest average daily egg production, followed by the TC genotype with medium egg production. Research by Cui et al. (2006) and Abdi et al. (2014) also stated that chickens with the CC genotype and C allele had better egg production and reproductive traits. Since this mutation point was in the intron position, and SNP g.8052T > C was in the exon position, the mutation points g.7835A > G and g.7886A > T were not associated with the characteristics of daily egg production in IPB-D1 chickens. Sakharkar et al. (2002) suggested that mutations in exons are part of a gene that will encode part of the mature RNA produced by a gene after the intron is removed during RNA splicing. Mutations in exons can affect polypeptide sequence, and mutations in introns can affect RNA processing and, hence, may influence the sequence and/or production of polypeptide (Krebs et al., 2017). The term of exon refers to the DNA sequence in a gene and the corresponding sequence in the RNA transcript. When the RNA splicing process occurs, the introns are removed, and the exons covalently combine with each other to produce the mature ambassador RNA.
Egg production in chickens is influenced by many factors. The broodstock selection is an effort that can be made to improve the genetic quality of the offspring and increase the number of eggs produced. One selection that can be used is the weight selection of the parent body. Lestari et al. (2020) stated that broodstocks with higher weight will experience peak production faster than lightweight broodstock due to variability and sexual maturity. This results in chickens with more weight producing faster, while chickens with less weight experiencing slower production. Untari et al. (2012) stated that several factors that can increase egg production include the selection of superior offspring, food consumed to meet energy needs and egg production, and good maintenance handling so as to prevent existing diseases from spreading quickly.
3.3 Association of SNP with body weight first on egg of chicken IPB-D1
The analysis of the association between each prolactin SNP gene with egg production or hen day production (HDP) of IPB-D1 chickens is presented in Table 3. Referring to Table 3, there was no association found at the three SNP points with the characteristics of the body weight first on egg in IPB-D1 chickens.
The value of the correlation coefficient between the nature of the body weight first on egg and the egg production of IPB-D1 chickens in this study was 14 % (r=0.144). However, there was no closeness of the association between the two variables (P > 0.05), so a correlation between the two characteristics was also not found. Harms et al. (1982) stated that the correlation between body weight first on egg and the number of eggs was negative. Jaelani et al. (2016) also mentioned that the initial laying of young hens with less weight takes longer because the nutrients obtained tend to be used to meet daily energy needs and growth. A correlation between the body weight first on egg and egg production has been found and, its potential is important to observe as a genetic marker for the marker-assisted selection (MAS) program.
Referring to Table 3, there was no association found at the three SNP points with the characteristics of the body weight first on egg in IPB-D1 chickens. This is because, in general, the performance of the prolactin gene does not directly and significantly control body weight characteristics. The prolactin gene, through its product, the prolactin hormone, specifically controls the variation in the number of eggs produced by reducing egg biosynthesis during the incubation period (Smiley, 2019). The prolactin promoter activates the prolactin gene's initial transcription, which causes this incubation to happen. If there is a mutation in the prolactin promoter, then the prolactin gene cannot work, so there is no incubation expression (Lumatauw and Mu'in, 2016). The body weight first on egg is significantly influenced by the growth hormone, which is a product of the chicken growth hormone (cGH) gene (Su et al., 2014).
It is crucial to understand whether there is a positive association between the initial egg body weight and egg production when considering this information as a selection criterion. As a result, information on the association between the SNP exon 5 prolactin gene and egg production traits, in addition to the positive correlation between body weight first on egg and egg production, may be used to carry out the broodstock selection process for female lines on IPB-D1 chicken. The three SNPs in this study were known not to be associated with the body weight first on egg in IPB-D1 chickens, so they could not be used as reference genetic marker candidates for the marker-assisted selection (MAS) program.
Three SNPs are found in the prolactin gene fragment exon 5 position in IPB-D1 chickens consisting of g.7835A > G, g.7886A > T, and g.8052T > C. The g.7835A > G is a synonymous mutation, while the g.7886A > T and g.8052T > C are non-synonymous mutations. All SNPs found in IPB-D1 chickens are polymorphic and are in Hardy–Weinberg equilibrium. The percentage of daily egg production in IPB-D1 hens is revealed to be significantly impacted by the study of the g.8052T > C mutation point association. When compared to other genotypes, genotype CC exhibited a greater proportion of hen day production due to the mutation g.8052T > C. The TC genotype exhibited medium egg output, whereas the TT genotype had the lowest average daily egg production. The study demonstrates that the prolactin gene may be considered to be a marker-assisted selection (MAS) candidate for the traits of IPB-D1 chicken egg production and to assist in the selection that will be implemented on IPB-D1 hens to produce new strains of female lines.
The data sets used in this article can be requested from the corresponding author.
LR was in charge of the experiments, carried out the data analysis, and wrote the original draft. SD, NU, and CS were responsible for the study design, data resources, and data validation. IK carried out the review and editing of the paper. All the authors read and approved the submitted version.
The contact author has declared that none of the authors has any competing interests.
This experiment did not damage the welfare of animals. All procedures carried out in this study have received approval from the Animal Care and Use Committee (ACUC) of IPB University (ACUC no. 163-2019 IPB). All efforts were made to minimize any discomfort during the experiment.
Publisher’s note: Copernicus Publications remains neutral with regard to jurisdictional claims in published maps and institutional affiliations.
We thank the Ministry of Research, Technology and Higher Education (Indonesia), which has financed this research through the collaboration of World Class Research.
This research has been supported by the Ministry of Research, Technology and Higher Education (Indonesia) (grant no. 121/SPH/LT/DRPM/2019) and Maching Fund Kedaireka (grant no. 15374/IT3.L2/HK.07.00/P/T/2022).
This paper was edited by Henry Reyer and reviewed by Karim El-Sabrout and lamiaa radwan.
Abdi, M., Seyedabadi, H., and Gorbani, A.: Prolactin and NPY gene polymorphism and its associations with production and reproductive traits in West-Azarbaijan native chicken, Bull. Env. Pharmacol. Life Sci., 6, 39–45, 2015.
Aldridge, M. N., Vandenplas, J., and Calus, M. P. L.: Efficient and accurate computation of base generation allele frequencies, J. Dairy Sci., 102, 1364–1373, https://doi.org/10.3168/jds.2018-15264, 2019.
Asiamah, A. C., Zou, K., Li Lu, L., Zhang, S. W., Xue, Y., Su, Y., and Zhao, Z.: Genetic effects of polymorphisms of candidate genes associated with ovary development and egg production traits in ducks, Anim. Reprod. Sci., 211, 106219, https://doi.org/10.1016/j.anireprosci.2019.106219, 2019.
Astuti, D. and Prijono, S. N.: Eggs production trait of Indonesian domestic ducks inferred from analysis of an SNP (single nucleotide analysis) genotypes in the 5th exon of prolactin gene, Proceeding The 7th ASIAHORCs General Meeting and the 5th Joint Symposium Bali, Bali, Indonesia, Indonesian Institute of Sciences (LIPI), 26–28 November 2013, 111–117, ISBN: 978-151-9349-64-8, 2014.
Bai, D. P., Hu, Y. Q., Li, Y. B., Huang, Z. B., and Li, A.: Polymorphisms of the prolactin gene and their association with egg production traits in two Chinese domestic ducks, Brit. Poultry Sci., 60, 125–129, https://doi.org/10.1080/00071668.2019.1567909, 2019.
Cui, J. X, Du, H. L., Liang, Y., Deny, X. M., Li, N., and Zhany, X. Q.: Association of polymorphism in the promoter region of chicken prolactin with age productio, Poult Sci., 85, 26–31, https://doi.org/10.1093/ps/85.1.26, 2006.
Dobolyi, A., Oláh, S., Keller, D., Kumari, R., Fazekas, E. A., Csikós, V., Renner, É., and Cservenák, M.: Secretion and Function of Pituitary Prolactin in Evolutionary Perspective, Front. Neurosci., 14, 621, https://doi.org/10.3389/fnins.2020.00621, 2020.
Erehehuara: Studies on the SNP of the chicken's prolactin gene, Huazhong Agricultural University, Wuhan, Ph.D. Thesis, Huazhong Agricultural University, 2003.
Gao, J., Xu, W., Zeng, T., Tian, Y., Wu, C., Liu, S., Zhao, Y., Zhou, S., Lin, X., Cao, H., and Lu, L.: Genome-wide association study of egg-laying traits and egg quality in lingkun chickens, Front. Vet. Sci., 20, 877739, https://doi.org/10.3389/fvets.2022.877739, 2022.
Goddard, M. E., Kemper, K. E., MacLeod, I. M., Chamberlain, A. J., and Hayes, B. J.: Genetics of complex traits: prediction of phenotype, identification of causal polymorphisms and genetic architecture, Proc. Biol. Sci., 283, 1835, https://doi.org/10.1098/rspb.2016.0569, 2016.
Guo, Y., Ye, F., Sheng, Q., Clark, T., and Samuels, D. C.: Three-stage quality control strategies for DNA re-sequencing data, Brief. Bioinform., 15, 879–889, https://doi.org/10.1093/bib/bbt069, 2014.
Habiburahman, R., Darwati, S., Sumantri, C., and Rukmiasih: Egg production and egg quality IPB D-1 Chicken G7 and repeatability estimation, J. Ilmu. Prod. dan. Teknol. Has Peternak., Jurnal Ilmu Produksi dan Teknologi Hasil Peternakan (JITEK), 8, 97–101, https://doi.org/10.29244/jipthp.8.2.97-101, 2020.
Harjosuwono, B. A., Arnata, I. W., and Puspawati, G. A. K. D.: Rancangan percobaan teori aplikasi SPSS dan excel, Lintas Kata Pub, Malang, ISBN: 978-602-99853-1-3, 2011.
Harms, R. H., Costa, T. P., and Miles, R. D.: Daily feed intake and performance of laying hens grouped according to body weight, Poult. Sci., 16, 1021–128, 1982.
Hartl, D. L. and Clark, A. G.: Principles of Population Genetics, Ed ke-3, Sinaeus Assosiatess Inc, Massachusetts, ISBN: 978-0878932726, 2000.
Jaelani, A., Widaningsih, N., and Rahmadi: Pengaruh umur induk dan produksi telur ayam parent stock, Media Sains., 9, 198–209, 2016.
Krebs, J. E., Goldstein, E. S., and Kilpatrick, S. T.: Lewin's GENES XII, Jones & Bartlett Learning, Massachusetts, ISBN: 9784449659852, 2017.
Kumar, S., Stecher, G., Li, M., Knyaz, C., and Tamura, K.: MEGA X: molecular evolutionary genetics analysis across computing platforms, Battistuzzi FU, Mol. Biol. Evol., 35, 1547–1549, https://doi.org/10.1093/molbev/msy096, 2018.
Lestari, M., Jan, T., Rozi, T., Kasip, L. M., and Muhsinin, M. A.: Study on characteristic of qualitative traits and morphometric of Kampung chickens with various comb-types in Lombok island, Jurnal Ilmu dan Teknologi Peternakan Indonesia, 6, 24–32, https://doi.org/10.29303/jitpi.v5i2.64, 2020.
Li, H. F., Shu, J. T., Du, Y. F., Shan, Y. J., Chen, K. W., Zhang, X. Y., Han W., and Xu, W. J.: Analysis of the genetic effects of prolactin gene polymorphisms on chicken egg production, Mol. Biol. Rep., 40, 289–294, https://doi.org/10.1007/s11033-012-2060-7, 2013.
Lukmanudin, M., Sumantri, C., and Darwati, S.: Body size of local chicken crossing IPB D-1 fifth-generation from age 2 until 12 weeks, Jurnal Ilmu Produksi dan Teknologi Hasil Peternakan, 6, 113–120, 2018.
Lumatauw, S. and Mu'in, M. A.: A 24-bp indel (insertion-deletion) polymorphism in promoter prolactin gene of papua local chickens, Animal Production, 18, 1–7, 2016.
Mazurowski, A., Frieske, A., Wilkanowska, A., Kokoszyński, D., Mroczkowski, S., Bernacki, Z., and Maiorano, G.: Polymorphism of prolactin gene and its association with growth and some biometrical traits in ducks, Ital. J. Animal Sci., 15, 200–206, https://doi.org/10.1080/1828051X.2016.1153405, 2016.
Nei, M. and Kumar, S.: Molecular Evolution and Phylogenetics, Oxford Univ Press, New York, ISBN: 9780195135848, 2000.
Noor, R. R.: Genetika Ternak, Penebar Swadaya, Jakarta, Indonesia, ISBN: 9794893404, 2010.
Sakharkar, M., Passetti, F., de Souza, J. E., Long, M., and de Souza, S. J.: Exint: an exon intron database, Nucl. Acid. Res., 30, 191–194, https://doi.org/10.1093/nar/30.1.191, 2002.
Shastry, B. S.: SNPs: impact on gene function and phenotype, Method. Mol. Biol., 578, 3–22, https://doi.org/10.1007/978-1-60327-411-1_1, 2017.
Smiley, K. O.: Prolactin and avian parental care: new insights and unanswered questions, Horm. Behav., 111, 114–130, https://doi.org/10.1016/j.yhbeh.2019.02.012, 2019.
Su, Y. J., Shu, J. T., Zhang, M., Zhang, X. Y., Shan, Y. J., Li, G. H., Yin, J. M., Song, W. T., Li, H. F., and Zhao, G. P.: Association of chicken growth hormone polymorphisms with egg production, Genet. Mol. Res., 13, 4893–903, https://doi.org/10.4238/2014.july.4.3, 2014.
Sumantri, C. and Darwati, S.: Pekembangan terkini riset ayam unggul IPB-D1, Prosiding Seminar Nasional Industri Peternakan 1, 3–7, Bogor, 29–30 November 2017, Institut Pertanian Bogor, Indonesia, ISBN: 978-602-96530-5-2, 2017.
Sumantri, C., Khaerunnisa, I., and Gunawan, A.: The genetic quality improvement of native and local chickens to increase production and meat quality in order to build the Indonesian chicken industry, IOP Conf. Ser.-Earth Environ. Sci., 492, 012099, https://doi.org/10.1088/1755-1315/492/1/012099, 2020.
Susanti, T.: Prolactin as a candidate gene controlling molting and egg production of duck, WARTAZOA, 25, 23–28, https://doi.org/10.14334/wartazoa.v25i1.1125, 2015.
Ulupi, N., Sumantri, C., and Darwati, S.: Resistance against Salmonella pullorum in IPB-D1 crossbreed, kampong and commercial broiler chicken, The 1st Conference Technology on Biosciences and Social Sciences, 88–91, Padang, 17–19 November 2016, Universitas Andalas, Padang, ISBN : 978-602-6381-22-4, 2016.
Untari, E. K. and Ismoyowati, S.: Perbedaan karakteristik tubuh ayam Kedu yang dipelihara kelompok tani ternak “Makukuhan Mandiri” di Temanggung, Jurnal Pembangunan Pedesaan, 13, 135–145, 2013.
Wang, C., Liang, Z., Yu, W., Feng, Y., Peng, X., Gong, Y., and Li, S.: Polymorphism of the prolactin gene and its association with egg production traits in native Chinese ducks, South Afr. J. Anim. Sci, 41, 63–69, https://doi.org/10.4314/sajas.v41i1.66044, 2011.
Weir, B. S.: Genetic Data Analysis II Methods for Discrete Population Genetic Data, Sinauer Associates Inc, Sunderland, 1196, ISBN: 9780878939022, 2011.
Wilkanowska, A., Mazurowski, A., Mroczkowski, S., and Kokoszyński, D.: Prolactin (PRL) and prolactin receptor (PRLR) genes and their role in poultry production traits, Folia Biol., 62, 1–8, https://doi.org/10.3409/fb62_1.1, 2014.
Zhang, L., Li, D. Y., Liu, Y. P., Wang, Y., Zhao, X. L., and Zhu, Q.: Genetic effect of the prolactin receptor gene on egg production traits in chickens, Genet. Mol. Res., 11, 4307–4315, https://doi.org/10.4238/2012.October.2.1, 2012.