the Creative Commons Attribution 4.0 License.
the Creative Commons Attribution 4.0 License.
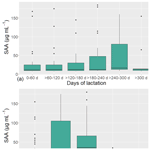
Changes of acute-phase proteins, glucose, and lipid metabolism during pregnancy in lactating dairy cows
Esterina Fazio
Arianna Bionda
Luigi Liotta
Annalisa Amato
Vincenzo Chiofalo
Paola Crepaldi
Katiuska Satué
Vincenzo Lopreiato
The study aimed to evaluate the effects of different stages of lactation (0 to > 300 d) and pregnancy (0 to > 180 d) on serum amyloid A (SAA), C-reactive protein (CRP), glucose, total cholesterol (TCho), and triglyceride (TG) concentrations in dairy cows of different breeds. Thus, 40 healthy multiparous cows (10 Holstein, 10 Simmental, 10 Brown, and 10 Modicana) were randomly selected, and blood samples were collected once every 60 d for 1 year. Overall, SAA and CRP serum concentrations progressively increased and became more variable along the lactation, peaking at > 240–300 d, and then decreased in the last period (> 300 d). Along pregnancy, SAA and CRP initially increased, with the highest concentrations at > 60–120 d, and then decreased until the last phase of pregnancy (> 180 d). However, lactation and gestation phases did not significantly affect SAA and CRP when all the cows were analyzed together. A significant and positive correlation was observed between SAA and CRP both along lactation (r=0.89; p<0.0001) and pregnancy (r=0.91; p<0.0001). Breeds only showed differences in CRP levels along gestation (p=0.0102), due to a peak registered at 0–60 d in Holstein cows. In pregnant cows, glucose was positively correlated with SAA (r=0.43; p=0.0017) and CRP (r=0.42; p=0.0019). Hence, these significant and positive relationships reflect the physiological adaptations of the dairy cows along both gestational and lactational dynamics, suggesting that these proteins may also be involved in non-pathological processes. In this perspective, this study established that the obtained response markedly varies among healthy individuals along lactation and gestation and thus that the physiological range of acute-phase proteins (APPs) is wide; this makes it difficult to use these proteins as a marker of different physiological reproductive and productive periods.
The acute-phase reaction represents a nonspecific response of the immune system, primarily started in reaction to inflammatory cytokines, with the synthesis of acute-phase proteins (APPs). These proteins are generally absent, or only found in traces, in healthy animals (Ceciliani et al., 2015). Recently, there has been a surge of interest regarding the clinical use of APPs in large ruminant medicine, both because they represent a sensitive and non-specific biomarker of infection, inflammation, or stress and because they are involved in the restoration of homeostasis (Grönlund et al., 2005; Petersen et al., 2004).
Serum amyloid A (SAA) is regarded as the major APP in most species, including domestic and wild ruminants (Eckersall and Bell, 2010; González et al., 2008; Miglio et al., 2013; O'Reilly and Eckersall, 2014; Rahman et al., 2010a, b). Stress and acute-phase protein response show an inconspicuous but essential linkage (Murata, 2007): in fact, an increase of these proteins was also observed in cattle subjected to physical stress (Alsemgeest et al., 1993), suggesting that the APP response is also activated under conditions unrelated to inflammation. Thereby, elevated serum SAA levels are also found in cows around peripartum, at parturition, peaking in the week following, probably due to delivery-related inflammatory status caused by stress or lesions at the genital apparatus, and then steadily decreasing during lactation (Humblet et al., 2006; Murata, 2007; Saremi et al., 2013). Thus, postpartum elevation of APPs can be associated with the birth process and/or factors in colostrum and not necessarily with disease-related processes (Orro et al., 2008). Hence, SAA has been shown to also be present in normal bovine colostrum and in the healthy bovine mammary gland (Kho et al., 2000; McDonald et al., 2001), and low concentrations of SAA and other APPs have often been demonstrated in plasma and milk of healthy cattle (Alsemgeest et al., 1994; Eckersall et al., 2001; Heegaard et al., 2000; Werling et al., 1996). The biological role of SAA remains mostly undisclosed, but several functions have been suggested, including a role in lipid transport and/or metabolism (Kisilevsky et al., 1996; Lindhorst et al., 1997). In fact, it has been shown that SAA derived from adipocytes acts as a mediator between hypertrophied adipocytes and macrophages by regulating the adipocyte cholesterol efflux (Poitou et al., 2009), suggesting that SAA may regulate adipogenesis, reorganize the adipose tissue, and enhance fatty acid oxidation by interacting with peroxisome proliferator-activated receptors (Schoonjans et al., 1996). Moreover, the presence of a mammary-specific SAA3 isoforms in colostrum hints that the protein may be involved in the non-pathological tissue remodeling during the lactational cycle and/or the adaptation of the newborn calf to extra-uterine life (Jacobsen et al., 2005).
Similarly to SAA, the C-reactive protein (CRP) has also been mentioned in various species (e.g., human or dog) as an important APP, receiving however limited interest in cattle (Petersen et al., 2004). The maintenance of homeostasis in the pregnant woman promotes a healthy pregnancy and normal adaptive processes in a developing fetus, and changes in CRP levels widely vary between subjects along pregnancy (Belo et al., 2005). Other factors have been related to increased CRP concentrations in women, such as advanced age, high parity, excessive body weight, and diet (Clendenen et al., 2011; Panagiotakos et al., 2005; Vasto et al., 2007), whereas in dairy cattle, body condition score, lactation status, and health but not ambient temperature, animal age, or parity showed a significant correlation (Lee et al., 2003).
In cows, the transition of pregnancy to lactation, with the concomitant negative energy balance during early lactation, requires substantial metabolic and physiological adaptations to guarantee a positive interaction between metabolism and fertility (Hachenberg et al., 2007; Leroy et al., 2008). In addition, a strong relationship between some variables of the energetic profile and acute-phase proteins in cows after parturition was recorded, where the acute-phase response occurring around parturition is associated with numerous changes in the lipid and glucose metabolism, such as decreased cholesterol, accelerated lipolysis, and increased non-esterified fatty acid plasma concentrations (Hardardóttir et al., 1994; Lopreiato et al., 2019, 2021; Minuti et al., 2020; Tóthová et al., 2014b).
We hypothesized that the presence of selected APPs and changes of some energy substrates might be related to physiological gestation and lactation phases. Therefore, the objectives of this study were (1) to assess the dynamics of changes in concentrations of serum amyloid A (SAA) and C-reactive protein (CRP), glucose, total cholesterol (TCho), and triglyceride (TG) at different stages of lactation and pregnancy in healthy dairy cows; and (2) to characterize the correlations between APPs and some energy substrates, according to the physiological period and breed.
2.1 Animals and diet
A total of 40 multiparous, clinically healthy dairy cows (10 Holstein, 10 Simmental, 10 Brown, and 10 Modicana) were randomly selected from the herd of a commercial farm located in Ragusa (36∘53′47′′ N, 14∘42′24.8′′ E; 500 m above sea level, Italy). The animals were homogeneous for age (4 ± 1.5 years), body condition score (3 ± 0.5), and lactation period (30 ± 20 d). Average milk production was 27 ± 3 kg per head per day for the Holstein, Brown, and Simmental breeds and 15 ± 2 kg per head per day for Modicana breed.
All animals were characterized by a normal cyclicity during the previous breeding seasons and absence of reproductive pathologies or inflammatory and infectious processes occurred and treated with antibiotics or anti-inflammatory within a month before the beginning of sampling.
The animals were raised according to the local tradition in the same environmental and managerial conditions. All cows were housed in a straw-bedded free-walking barn, equipped with automatic system fans and sprinklers that were activated during the hot season; pasture access was allowed in spring and autumn (for a minimum of 6 h during daylight, from 08:00 to 14:00 CEST) but not in summer. All cows were milked twice daily at 04:30 and 16:30 CEST. The same diet was fed throughout the entire trial period and was provided as a total mixed ration (TMR) twice a day at 08:00 and 14:00 CEST; it was composed of meadow hay and concentrate (20 kg of dry matter (DM) per head), which was supplemented with 8 % of dried and pitted olive cakes (DM: 95.6 %; on DM basis: 10.4 % of crude protein, 15.9 % of ether extract, 49.4 % of neutral detergent fiber, 39.4 % of acid detergent fiber, 23.1 % of acid detergent lignin, 3.87 % of ash, and 1.57 % of starch), according to an approved UE disciplinary called “QS Sicilia” aimed at the recovery of agro-industrial by-products.
Blood samples were collected from all the enrolled cows once every 60 d, for a year (February 2021–February 2022), at different stages of lactation (0–60; > 60–120, > 120–180, > 180–240, > 240–300, and > 300 d, which corresponded to an average number of days equal to 34, 87, 155, 241, 269, and 339, respectively, with a standard deviation of 20 d) and pregnancy (0–60 d, > 60–120, > 120–180, and > 180 d, which corresponded to an average number of days equal to 27, 89, 157, and 225, respectively, with a standard deviation of 20 d). The day of calving was considered day 0 of gestation and the last day of pregnancy. Day 0 of pregnancy was assessed using the registration of the insemination days and the day of calving, considering an average gestation duration of 285 d in cows. Blood samples (10 mL) were collected by vacuum blood collection tubes before feeding in the morning (07:00 CEST) from the jugular vein of each cow and then centrifuged at 2000×g for 10 min; the separated supernatant serum was collected and stored at −20 ∘C for later analyses. At the time of each sampling, all the animals were examined by a veterinarian, and the following biochemical parameters were evaluated by an enzymatic colorimetric method, using automated spectrophotometry (Biotecnica Instruments BT 3500): liver (total bilirubin, aspartate aminotransferase, alanine amino transferase, lactate dehydrogenase), renal (urea), and muscular (creatine kinase) parameters. The results of biochemistry profile were evaluated to test and verify the good health status of all dairy cows used in this study.
2.2 Determination of serum amyloid type A (SAA), C-reactive protein (CRP), glucose, TCho, and TG concentrations
SAA concentration (µg mL−1) was analyzed by the method of competitive sandwich enzyme-linked immunosorbent assay using a commercial OmniChek™ – SAA kit no. R1070818 (Agrolabo spa, Turin, Italy) in microplates. The intra- and inter-assay coefficients of variability (CVs) were 3.1 %–6.4 % and 5.9 %–11.2 %, respectively. Medical system sensitivity was < 10 µg mL−1.
The CRP (ng mL−1) concentration was determined using an automated Sandwich immunoluminometric assay, adapted to an automatic analyzer Maglumi 800 (CRP CLIA, Lotus Global Co., Ltd, London, UK, kit no. 130216002M). The intra- and inter-assay CVs were 5.3 %–7.7 % and 7.0 %–12.5 %, respectively. Medical system sensitivity was typically less than 0.13 ng mL−1.
Glucose concentration was analyzed by automated spectrophotometry (Biotecnica Instruments BT 3500) using the colorimetric enzymatic method GOD-POD (kit no. 050720). Triglycerides and total cholesterol concentrations were analyzed by enzymatic colorimetric method, using the BT 3500 (Biotecnica Instruments; kit nos. 020721 and 010321, respectively).
2.3 Statistical analysis
Statistical analyses were carried out using JMP® 16 (SAS Institute Inc., Cary, NC). Descriptive statistics was obtained for all the studied parameters. The effect of the pregnancy or lactation phase was assessed with analysis of variance (ANOVA) or the nonparametric Kruskal–Wallis test, in the case of deviation from normality. In addition, a two-way ANOVA with interaction was applied to assess the effect of breed, pregnancy, or lactation phase and their interaction on SAA and CRP concentrations. Multiple comparisons were performed using Kruskal–Wallis or Steel–Dwass post hoc tests, respectively. The correlation between the parameters was expressed using Pearson's coefficient (r). Differences were considered to be statistically significant when p<0.05.
Figure 1 reports the overall blood SAA and CRP concentrations along lactation (0 to > 300 d) and pregnancy (0 to > 180 d) in all the enrolled dairy cows.
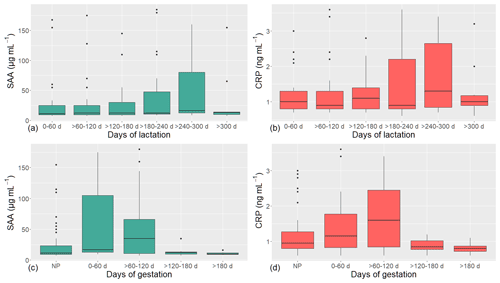
Figure 1Box plots representing the measured serum concentrations of serum amyloid-A (SAA) and C-reactive protein (CRP) along lactation and gestation in dairy cows (n=40). NP – nonpregnant.
The first days postpartum represent a very critical period for cows; therefore, with regard to the first lactation phase (0–60 d), we compared the measurements of the cows in the first 2 weeks postpartum and in the rest of the phase, before performing the following analyses. No difference for SAA, CRP, glucose, cholesterol, or triglyceride was found (p=0.8603, 0.4266, 0.9547, 0.8361, and 0.6379, respectively). Therefore, in order to have groups homogeneous in size, we aggregated the measurement of the cows in the first 60 d of lactation.
Serum SAA and CRP concentrations showed a constant pattern from 0–60 to > 120–180 d of lactation, then an increase, peaking at > 240–300, and lastly a decrease until the end of lactation (Fig. 1a, b). However, it should be noted that no significant differences were found among the different phases (p=0.4043 for SAA and 0.9464 for CRP). The mean concentration of SAA recorded along lactation was 34.65 µg mL−1, and the individual values ranged from 7.00 to 185.00 µg mL−1. The mean CRP concentration in dairy cows was 1.36 ng mL−1, with values ranging from 0.60 to 3.60 ng mL−1.
When breed, lactation stage, and their interaction were all used as factors, no significant effects were observed. However, a similar SAA and CRP trend was observed along the lactation for Simmental and Modicana cows, which showed the highest values at > 240–300 d and then a decrease in the last phase; conversely, in Brown cows they steadily increased from > 120–180 d to the end of lactation, whereas in Holsteins the concentrations of APPs peaked at > 60–120 and then at > 180–240 d (Table 1).
Table 1Least-square means, standard error (SEM), and p values relative to the effect of breed, lactation phase, and their interaction on serum amyloid A (SAA) and C-reactive protein (CRP) (n=40).
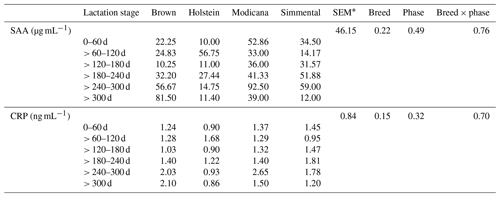
* Greatest standard error of the mean.
Regarding the energy substrates, serum glucose levels increased along the lactation (p=0.0002), with the first two periods (0–120 d) showing significantly higher values than the last two (> 120 d). Instead, both TCho and TG concentrations showed a progressive increase from 0–60 d to 240–300 d, which registered the highest values, and then a tendency to decrease in the last phase of lactation (Table 2). However, only TCho showed significant differences among the phases (p=0.0190), particularly between 0–60 and > 240–300 d. A significant and positive correlation between TCho and TG along lactation (r=0.31; p<0.0005) and SAA and CRP (r=0.91; p<0.0001) was recoded. The pairwise correlation between all the other parameters, instead, was not significant.
Table 2Mean ± standard deviation of glucose, cholesterol, and triglyceride serum concentrations in lactating dairy cows (n=40). The p values refer to the effect of lactation stage on the parameters; within each row, different superimposed letters indicate significant differences between lactation periods.

Along the whole pregnancy, serum SAA concentrations were highly variable in the first months (0–120 d), whereas in the second part of the gestation they tended to decrease (Fig. 1c). Serum CRP concentrations showed a biphasic trend, increasing from 0–60 to > 60–120 d and then consistently decreasing until the end of pregnancy (Fig. 1d). However, the gestation phases did not significantly affect SAA and CRP concentrations (p=0.1223 and 0.1482, respectively). Overall, the concentration of SAA recorded along gestation in dairy cows was on average 39.49 µg mL−1, with values ranging from 7.00 to 180.00 µg mL−1. The average CRP concentration was 1.36 ng mL−1, and the values ranged from 0.60 to 3.60 ng mL−1. SAA and CRP were positively correlated in this phase as well (r=0.91; p<0.0001). No significant differences were observed between nonpregnant and pregnant (i.e., all considering together the cows in all the gestation phases) dairy cows either. When the effects of breed, gestation stage, and their interaction were analyzed together, a significant difference in SAA and CRP concentration was observed according to both the gestation phase and its interaction with breed, whereas the breed effect was significant for CRP concentration only (Table 3). In particular, Holstein showed higher CRP values, probably due to the peak registered at 0–60 d of pregnancy. In all the breeds, serum SAA and CRP concentrations showed a biphasic trend: Modicana cows showed a peak of both SAA and CRP at 0–60 d as well, whereas Brown and Simmental did at > 60–120 d; in all the breeds (except Modicana, which could not be sampled in this phase), these proteins tended to decrease in the last phase of pregnancy (> 180 d) (Table 3). In any case, SAA and CRP measurement showed a high intra- and inter-individual variability along gestation.
Table 3Least-square means, standard error (SEM), and p values relative to the effect of breed, gestation phase, and their interaction on serum amyloid A (SAA) and C-reactive protein (CRP) (n=30).
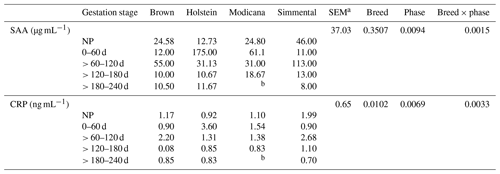
a Greatest standard error of the mean. b For managerial reasons and due to a later insemination compared to the other breeds, it was not possible to have data of Modicana cows in this phase.
Even though differences among phases were not significant (p=0.0732), it should be noted that serum glucose concentration trend almost paralleled that of SAA and CRP along pregnancy, lightly increasing from 0–60 to > 60–120 d and then decreasing until the end of gestation (Table 4). Indeed, pregnant dairy cows showed significant and positive correlations between glucose and both SAA (r=0.43; p<0.0017) and CRP (r=0.42; p<0.0019). Serum TCho and TG concentrations tended to increase with the advance of pregnancy until the end, with particularly high values for TG at > 180 d but no significant differences among the phases (p=0.1615) (Table 4).
4.1 Lactation
During the transition period, it is well known that dairy cows can experience a markedly variable status of systemic inflammation, even without clear signs of infection or any pathological condition (Bradford et al., 2015; Lopreiato et al., 2020). The increase in the APP concentration around calving supports the concept that an inflammatory reaction is a physiological epiphenomenon of the onset of lactation that is independent of metabolic stress (Sauerwein et al., 2020).
Nevertheless, data obtained in this study show that, despite the involvement of cells and inflammatory mediators at the beginning of lactation and galactopoiesis (Sauerwein et al., 2020), different lactating phases seem not to affect the systemic SAA and CRP responses in dairy cows significantly. Moreover, the trend of CRP is in line with the dynamics previously described in cattle, in which CRP increases along lactation in parallel with milk production, peaking during high lactation, and decreases when lactation ceases. In this sense, it is possible to presume that bovine CRP concentrations may be lactation-associated rather than synthesized in the liver, as previously observed by Morimatsu et al. (1991). In fact, the lack of significant changes of their concentrations along the lactation indicates that dairy cows can sustain a physiological reaction, maintaining variable yet normal SAA and CRP ranges, independently of presumed metabolic stress of this period. The wide range and high standard deviation of measured SAA and CRP concentrations reflect the highest inter-individual variability of each animal to different physiological periods, as previously recorded by Tóthová et al. (2014a), confirming that the synthesis of APPs varies not only in different physiological periods, but also among and within different animal species (Pyörälä, 2000). In addition, it is well known that in ruminants SAA concentrations can reach rapidly up to a 10- to 100-fold increase (major APP) or up to a 2- to 10-fold increase (moderate APP) over the baseline value in response to stimuli, whereas CRP concentrations can reach rapidly up to a 2- to 10-fold increase (moderate APP) (Heegaard et al., 2000; Murata et al., 2004).
Data obtained showed that, in our experimental conditions, the physiological APP ranges recorded could be the expression of healthy cows, which is the most essential requirement for their welfare. Our data, therefore, confirm that animals may react to the metabolic changes occurring in this delicate transition period with a set of physiological adjustments, including variation in the concentration of some selected acute-phase proteins and energy substrates, as confirmed by the positive correlation observed between SAA and CRP and between TCho and TG along the whole lactation period.
In addition, the lower serum tCho concentrations in the early lactation phase were presumably attributed to the moderately increased lipoprotein lipase activity, occurring alongside the mammary lipogenic enzyme activation to provide milk fat biosynthesis, as recently described also in goats (Liotta et al., 2021). Indeed, according to previous studies, milk fat percentages are highest in colostrum and then decline during the first 2 months of lactation and finally slowly increase as it progresses (Linn, 1988). Milk fat derives both from preformed fatty acids from the blood and mammary gland synthesis from acetate and beta-hydroxybutyrate (Chilliard et al., 2000; Shingfield et al., 2010), both of which reduce blood levels of total cholesterol and triglycerides.
4.2 Gestation
In human medicine, physiological reactions around parturition are known to trigger several key events that promote uncontrolled systemic inflammation (Gatzka et al., 2002; Sordillo et al., 2009), contributing to the disruption of homeostasis. Although similar changes in the homeostasis during the periparturient period were observed in dairy cows, the physiopathology of these events in the immune functions is less clear (Tóthová et al., 2014b). The immune system is known to be considerably affected during pregnancy: its significant interactions with reproductive activities are critical for the maintenance of pregnancy but are also responsible for the immune suppression that is associated with an increased risk of diseases (Kehrli et al., 2006; Lippolis, 2008). An increased myometrial activity and uterine involution and regeneration are generally responsible for the higher circulating APP concentration around the time of parturition (Krakowski and Zdzisinska, 2007). The increase in concentration of the APP around calving supports the concept that an inflammatory reaction is a physiological epiphenomenon of the onset of lactation that is independent of metabolic stress (Sauerwein et al., 2020).
Nevertheless, the absence of significant changes in the profile of SAA between nonpregnant and pregnant dairy cows of this study is in line with the results previously obtained in bitches (Ulutas et al., 2009), sheep (Albay et al., 2014), and mares (Satué et al., 2021).
On the other hand, the results recorded in women and goats partially confirm those obtained in cows, in which the highest SAA values were obtained only in the second (> 60–120 d) and third (> 120–180 d) 2-month period of gestation. Indeed, in goats, SAA concentrations increase significantly in the second month of gestation and remain elevated until the end of pregnancy, with a peak at kidding (Czopowicz et al., 2017). In pregnant women, SAA is expressed in the trophoblast in the first trimester (Kovacevic et al., 2006) and in placental cells in the third trimester (Sandri et al., 2014), suggesting that this protein may have a consistent role along the progression of gestation, which, in fact, can be considered a state of mild systemic inflammation mediated also by the cytokines derived from placenta and extraplacental membranes.
We observed the absence of significant changes in CRP levels measured during the gestation in dairy cows, with a progressive and stable decrease throughout the last stage. Likewise, according to our results, pregnant women also showed markedly different CRP trend during pregnancy, thus making its changes throughout the different phases of gestation not significant (Belo et al., 2005). Moreover, the trend we recorded is in accordance with the dynamics previously described in Thoroughbred mares, in which this protein decreased during the last 4 months of gestation, reaching minimum values 2 months before foaling (Yamashita et al., 1991), and in cattle, in which CRP increases along lactation in parallel with milk production, peaking during high lactation (corresponding to 2–4 months of pregnancy), and decreases when lactation ceases, supporting the hypothesis that lactation might stimulate CRP synthesis in bovine liver as an acute-phase reaction (Lee et al., 2003; Morimatsu et al., 1991).
Heikkinen et al. (2003) showed that in the sixth week of pregnancy, in humans, there is a substantial rise in the number of monocytes and macrophages in the systemic and placental circulation. These cells promote the trophoblastic development (Heikkinen et al., 2003) synthesizing IL-6, which is also the primary stimulus for CRP production (Diehl and Rincón, 2002). In cows, placentomes are present at 40–60 d, and the placenta expands during the first half of gestation (Reynolds et al., 2005).
Sacks et al. (1998) recorded different SAA and CRP concentrations in pregnant and nonpregnant cows, due to different hormone levels and/or modifications of the inflammatory response in normal gestations; however, no significant differences were obtained comparing nonpregnant and pregnant dairy cows in this study.
Another finding of interest was that the gestation phase showed a significant effect on SAA and CRP concentrations when analyzed together with the breed and that breed itself had a significant effect on CRP concentration only. Thus, the breed may play an unknown but physiologically important role during the different pregnant phases, probably due to the innate (endocrine and inflammatory) differences that contributed to guarantee of homeostatic responsiveness. In any case, it should be underlined that, from a practical standpoint, SAA and CRP measurements also showed a high intra- and inter-individual variability along gestation, probably, at least in part, due to the small number of enrolled animals.
Hence, it is possible to conclude that the changes of CRP and SAA along the different gestation phases in dairy cows could contribute to guaranteeing an alternative means to regulate the adaptive response that maintains a healthy pregnancy status. However, the existence of contradictory results indicates that further studies are needed to elucidate the emerging role of APPs as a marker of welfare in dairy cows and clarify the interrelationship of APPs and physiological processes that occur during pregnancy.
Related to the metabolic response, it is then reasonable to assume that at the end of pregnancy and in early lactation, all dairy cows transiently experience a decreased response to insulin in the peripheral tissues, to preserve a sufficient glucose supply for the fast-growing fetus and the mammary gland (De Koster and Opsomer, 2013). This evidence could suggest that these tissues drain most of the available glucose at these stages, as confirmed by the lowest values recorded at the end of lactation and gestation. In addition, the evidence that the serum glucose concentration trend almost paralleled that of SAA and CRP and its significant and positive relationships with SAA and CRP along pregnancy confirm that pregnancy depends on glucose as a substrate for tissue synthesis and metabolic energy and on the immune function, like acute-phase protein's mediators, for potential benefits in dairy cattle (Lucy, 2016). This trend showed that glucose can be considered a coordinator of the endocrine mechanisms controlling homeorhesis at the initiation of lactation (Lucy et al., 2014) and that the sum of the affected mechanisms can impinge upon the cow's immune system, perhaps influencing postpartum health by affecting immune cells that fight common postpartum diseases (Moyes, 2015).
The lipidic trend of pregnant dairy cows, characterized by the lowest values at the beginning of pregnancy, with a progressive tendency to increase along other periods until the end, even though not significant, is in line with the significant increase of serum total cholesterol and triglyceride concentrations described during the last gestational phase in small ruminants (Liotta et al., 2021; Waziri et al., 2010), which was attributed to an increased hepatic triglyceride synthesis (Okonkwo et al., 2010; Sharma et al., 2015; Tharwat et al., 2013; Waziri et al., 2010).
In dairy cows, pregnancy and lactation imply comprehensive functional and hormonal changes, including a systemic inflammatory reaction and oxidative stress around calving. Our observations may have a practical application, showing that an increase of SAA or CRP concentrations during pregnancy and lactation may not necessarily warn clinicians about severe diseases, such as metritis and endometritis, mastitis, and other inflammatory conditions.
Thus, changes of the selected APPs during different physiological periods may play a still unknown but physiologically important role in the adaptations of the dairy cows, according to their intra- and inter-individual variability. However, given the highly variable individual responses obtained, the SAA and CRP physiological range seems to be rather wide, making it difficult to use their concentration as a marker of different physiological reproductive and productive periods. For these reasons, the authors confirm that their interpretation should take into account other more specific markers that have been shown to be correlated to these proteins and reflect the temporal and physiological adaptation of the dairy cows along both gestational and lactational dynamics.
Although it still remains unanswered how the APPs' ubiquitous effects may limit their use in veterinary topics, it is certain that raised APP concentrations can have health-related implications for dairy cows. Hence, future applications of APP analyses should not be restricted to their current use to identify animal disease. Indeed, APPs might be measured to help to monitor the health and welfare of livestock, improving their productive and reproductive performance.
In this perspective, this study established reference values for SAA and CRP in all pregnancy phases and along lactation in different cow breeds.
The datasets generated during and/or analyzed during the current study are available from the corresponding author on reasonable request.
Conceptualization was carried out by EF and LL. AB and VL were responsible for validation. Formal analysis was carried out by AB. VC was responsible for methodology. Investigation was done by VL and AA. AB, PC, and VL were responsible for data curation. EF wrote the original draft. EF, AB, and VL reviewed and edited the text. AB, PM, KS, and LL were responsible for visualization. LL supervised the work, administered the project, and acquired funding. All authors read and agreed to the published version of the paper.
The contact author has declared that none of the authors has any competing interests.
The experimental protocol was approved by the Ethical committee of the Department of Veterinary Science of the University of Messina, Italy (code 041/2020). The research complied with guidelines of good clinical practices (EMEA, 2000). This study was performed according to the Italian and European regulations on animal welfare (Directive 2010/63/EU of the European Parliament and of the Council of 22 September 2010 on the protection of animals used for scientific purposes).
Publisher’s note: Copernicus Publications remains neutral with regard to jurisdictional claims in published maps and institutional affiliations.
The authors would like to thank Lissandrello farm (Ragusa, Italy) guesting the field trials and the feed industry Mangimi Di Pasquale (Avola, Italy) for concentrate production and supply.
This research has been supported by the P.O. FESR SICILIA 2014/2020: Obiettivo Tematico 1 – Ricerca, Sviluppo Tecnologico e Innovazione Obiettivo specifico; 1.1 – Incremento dell’attività di innovazione delle imprese Azione; 1.1.5 – Sostegno all’avanzamento tecnologico delle imprese attraverso il finanziamento di linee pilota e azioni di validazione precoce dei prodotti e di dimostrazione su larga scala; project BIOTRAK (grant no. 08SR1091000150-CUP G69J18001000007) (principal investigator Luigi Liotta).
This paper was edited by Joachim Weitzel and reviewed by two anonymous referees.
Albay, M., Karakurum, M., Sahinduran, S., Sezer, K., Yildiz, R., and Buyukoglu, T.: Selected serum biochemical parameters and acute phase protein levels in a herd of Saanen goats showing signs of pregnancy toxaemia, Vet. Med., 59, 336–342, https://doi.org/10.17221/7620-VETMED, 2014.
Alsemgeest, S. P., Taverne, M. A., Boosman, R., van der Weyden, B. C., and Gruys, E.: Peripartum acute-phase protein serum amyloid-A concentration in plasma of cows and fetuses, Am. J. Vet. Res., 54, 164–167, 1993.
Alsemgeest, S. P., Kalsbeek, H. C., Wensing, T., Koeman, J. P., van Ederen, A. M., and Gruys, E.: Concentrations of serum amyloid-A (SAA) and haptoglobin (HP) as parameters of inflammatory diseases in cattle, Vet. Q., 16, 21–23, https://doi.org/10.1080/01652176.1994.9694410, 1994.
Belo, L., Santos-Silva, A., Rocha, S., Caslake, M., Cooney, J., Pereira-Leite, L., Quintanilha, A., and Rebelo, I.: Fluctuations in C-reactive protein concentration and neutrophil activation during normal human pregnancy, Eur. J. Obstet. Gynecol. Reprod. Biol., 123, 46–51, https://doi.org/10.1016/j.ejogrb.2005.02.022, 2005.
Bradford, B. J., Yuan, K., Farney, J. K., Mamedova, L. K., and Carpenter, A. J.: Invited review: Inflammation during the transition to lactation: New adventures with an old flame, J. Dairy Sci., 98, 6631–6650, https://doi.org/10.3168/jds.2015-9683, 2015.
Ceciliani, F., Soler, L., Grilli, G., Marques, A. T., Giudice, C., and Lecchi, C.: The localization and differential expression of Serum Amyloid A in bovine liver and adipose tissue depots, Vet. Immunol. Immunopathol., 168, 35–39, https://doi.org/10.1016/j.vetimm.2015.08.004, 2015.
Chilliard, Y., Ferlay, A., Mansbridge, R. M., and Doreau, M.: Ruminant milk fat plasticity: nutritional control of saturated, polyunsaturated, trans and conjugated fatty acids, Ann. Zootech., 49, 181–205, https://doi.org/10.1051/animres:2000117, 2000.
Clendenen, T. V, Koenig, K. L., Arslan, A. A., Lukanova, A., Berrino, F., Gu, Y., Hallmans, G., Idahl, A., Krogh, V., Lokshin, A. E., Lundin, E., Muti, P., Marrangoni, A., Nolen, B. M., Ohlson, N., Shore, R. E., Sieri, S., and Zeleniuch-Jacquotte, A.: Factors associated with inflammation markers, a cross-sectional analysis, Cytokine, 56, 769–778, https://doi.org/10.1016/j.cyto.2011.09.013, 2011.
Czopowicz, M., Szaluś-Jordanow, O., Mickiewicz, M., Witkowski, L., Markowska-Daniel, I., Stefaniak, T., Reczyńska, D., Bagnicka, E., and Kaba, J.: Acute-phase proteins in pregnant goats: a longitudinal study, J. Vet. Diagn. Invest., 29, 814–819, https://doi.org/10.1177/1040638717714295, 2017.
De Koster, J. D. and Opsomer, G.: Insulin Resistance in Dairy Cows, Vet. Clin. North Am. Food Anim. Pract., 29, 299–322, https://doi.org/10.1016/J.CVFA.2013.04.002, 2013.
Diehl, S. and Rincón, M.: The two faces of IL-6 on Th1 Th2 differentiation, Mol. Immunol., 39, 531–536, https://doi.org/10.1016/s0161-5890(02)00210-9, 2002.
Directive 2010/63/EU of the European Parliament and of the Council of 22 September 2010 on the protection of animals used for scientific purposes (Text with EEA relevance), OJ, L276, 33–79, https://eur-lex.europa.eu/legal-content/EN/TXT/PDF/?uri=CELEX:32010L0063&from=EN (last access: 18 May 2021), 2010.
Eckersall, P. D. and Bell, R.: Acute phase proteins: Biomarkers of infection and inflammation in veterinary medicine, Vet. J., 185, 23–27, https://doi.org/10.1016/j.tvjl.2010.04.009, 2010.
Eckersall, P. D., Young, F. J., McComb, C., Hogarth, C. J., Safi, S., Weber, A., McDonald, T., Nolan, A. M., and Fitzpatrick, J. L.: Acute phase proteins in serum and milk from dairy cows with clinical mastitis, Vet. Rec., 148, 35–41, https://doi.org/10.1136/vr.148.2.35, 2001.
EMEA: VICH GL9: Good clinical practice, 1–27, https://www.ema.europa.eu/en/documents/scientific-guideline/vich-gl9-good-clinical-practices-step-7_en.pdf (accessed 10 August 2022), 2000.
Gatzka, C., Bremerich, D., Kaufmann, M., and Ahr, A.: Isolated decrease of haptoglobin during pregnancy: diagnosis by chance or pathological?, Zentralbl. Gynakol., 124, 120–122, https://doi.org/10.1055/s-2002-24234, 2002.
González, F. H. D., Tecles, F., Martínez-Subiela, S., Tvarijonaviciute, A., Soler, L., and Cerón, J. J.: Acute phase protein response in goats, J. Vet. Diagn. Invest., 20, 580–584, https://doi.org/10.1177/104063870802000507, 2008.
Grönlund, U., Hallén Sandgren, C., and Persson Waller, K.: Haptoglobin and serum amyloid A in milk from dairy cows with chronic sub-clinical mastitis, Vet. Res., 36, 191–8, https://doi.org/10.1051/vetres:2004063, 2005.
Hachenberg, S., Weinkauf, C., Hiss, S., and Sauerwein, H.: Evaluation of classification modes potentially suitable to identify metabolic stress in healthy dairy cows during the peripartal period, J. Anim. Sci., 85, 1923–1932, https://doi.org/10.2527/jas.2006-480, 2007.
Hardardóttir, I., Grünfeld, C., and Feingold, K. R.: Effects of endotoxin and cytokines on lipid metabolism, Curr. Opin. Lipidol., 5, 207–215, https://doi.org/10.1097/00041433-199405030-00008, 1994.
Heegaard, P. M., Godson, D. L., Toussaint, M. J., Tjørnehøj, K., Larsen, L. E., Viuff, B., and Rønsholt, L.: The acute phase response of haptoglobin and serum amyloid A (SAA) in cattle undergoing experimental infection with bovine respiratory syncytial virus, Vet. Immunol. Immunopathol., 77, 151–159, https://doi.org/10.1016/s0165-2427(00)00226-9, 2000.
Heikkinen, J., Möttönen, M., Komi, J., Alanen, A., and Lassila, O.: Phenotypic characterization of human decidual macrophages, Clin. Exp. Immunol., 131, 498–505, https://doi.org/10.1046/j.1365-2249.2003.02092.x, 2003.
Humblet, M.-F., Guyot, H., Boudry, B., Mbayahi, F., Hanzen, C., Rollin, F., and Godeau, J.-M.: Relationship between haptoglobin, serum amyloid A, and clinical status in a survey of dairy herds during a 6-month period, Vet. Clin. Pathol., 35, 188–193, https://doi.org/10.1111/j.1939-165x.2006.tb00112.x, 2006.
Jacobsen, S., Niewold, T. A., Kornalijnslijper, E., Toussaint, M. J. M., and Gruys, E.: Kinetics of local and systemic isoforms of serum amyloid A in bovine mastitic milk, Vet. Immunol. Immunopathol., 104, 21–31, https://doi.org/10.1016/j.vetimm.2004.09.031, 2005.
Kehrli, M. E., Neill, J. D., Burvenich, C., Goff, J. P., Lippolis, J. D., Reinhardt, T. A., and Nonnecke, B. J.: Energy and protein effects on the immune system, in: Ruminant physiology. Digestion, metabolism and impact of nutrition on gene expression, immunology and stress, edited by: Sejrsen, K., Hvelplund, T., and Nielsen, M. O., Wageningen Academic Publishers, the Netherlands, ISBN: 978-90-76998-64-0 2006.
Kho, Y. J., Cho, K. K., Kim, S. C., Kim, S. H., Chung, M. I., Baik, M. G., and Choi, Y. J.: Rapid communication: cloning of bovine serum amyloid A3 cDNA, J. Anim. Sci., 78, 2756, https://doi.org/10.2527/2000.78102756x, 2000.
Kisilevsky, R., Lindhorst, E., Ancsin, J. B., Young, D., and Bagshaw, W.: Acute phase serum amyloid A (SAA) and cholesterol transport during acute inflammation: A hypothesis, Amyloid, 3, 252–260, https://doi.org/10.3109/13506129609014373, 1996.
Kovacevic, A., Hammer, A., Sundl, M., Pfister, B., Hrzenjak, A., Ray, A., Ray, B. K., Sattler, W., and Malle, E.: Expression of serum amyloid A transcripts in human trophoblast and fetal-derived trophoblast-like choriocarcinoma cells, FEBS Lett., 580, 161–167, https://doi.org/10.1016/j.febslet.2005.11.067, 2006.
Krakowski, L. and Zdzisinska, B.: Selected cytokines and acute phase proteins in heifers during the ovarian cycle course and in different pregnancy periods, Bull. Vet. Inst. Puławy, 51, 31–36, 2007.
Lee, W.-C., Hsiao, H.-C., Wu, Y.-L., Lin, J.-H., Lee, Y.-P., Fung, H.-P., Chen, H.-H., Chen, Y.-H., and Chu, R.-M.: Serum C-reactive protein in dairy herds, Can. J. Vet. Res., 67, 102–107, 2003.
Leroy, J. L. M. R., Vanholder, T., Van Knegsel, A. T. M., Garcia-Ispierto, I., and Bols, P. E. J.: Nutrient prioritization in dairy cows early postpartum: mismatch between metabolism and fertility?, Reprod. Domest. Anim., 43, 96–103, https://doi.org/10.1111/j.1439-0531.2008.01148.x, 2008.
Lindhorst, E., Young, D., Bagshaw, W., Hyland, M., and Kisilevsky, R.: Acute inflammation, acute phase serum amyloid A and cholesterol metabolism in the mouse, Biochim. Biophys. Acta, 1339, 143–154, https://doi.org/10.1016/s0167-4838(96)00227-0, 1997.
Linn, J. G.: Factors affecting the composition of milk from Dairy Cows, in Designing Foods: Animal Product Options in the Marketplace, edited by National Research Council (US) Committee on Technological Options to Improve the Nutritional Attributes of Animal Products, National Academies Press (US), Washington, ISBN: 978-0-309-03795-2, https://doi.org/10.17226/1036, 1988.
Liotta, L., Bionda, A., Quartuccio, M., De Nardo, F., Visalli, R., and Fazio, E.: Thyroid and lipidic profiles in nicastrese goats (Capra hircus) during pregnancy and postpartum period, Animals, 11, 2386, https://doi.org/10.3390/ani11082386, 2021.
Lippolis, J. D.: Immunological signaling networks: integrating the body's immune response, J. Anim. Sci., 86, E53–E63, https://doi.org/10.2527/jas.2007-0620, 2008.
Lopreiato, V., Minuti, A., Trimboli, F., Britti, D., Morittu, V. M., Cappelli, F. P., Loor, J. J., and Trevisi, E.: Immunometabolic status and productive performance differences between periparturient Simmental and Holstein dairy cows in response to pegbovigrastim, J. Dairy Sci., 102, 9312–9327, https://doi.org/10.3168/jds.2019-16323, 2019.
Lopreiato, V., Mezzetti, M., Cattaneo, L., Ferronato, G., Minuti, A., and Trevisi, E.: Role of nutraceuticals during the transition period of dairy cows: a review, J. Anim. Sci. Biotechnol., 11, 96, https://doi.org/10.1186/s40104-020-00501-x, 2020.
Lopreiato, V., H. Ghaffari, M., Cattaneo, L., Ferronato, G., Alharthi, A. S., Piccioli-Cappelli, F., Loor, J. J., Trevisi, E., and Minuti, A.: Suitability of rumination time during the first week after calving for detecting metabolic status and lactation performance in simmental dairy cows: a cluster-analytic approach, Ital. J. Anim. Sci., 20, 1909–1923, https://doi.org/10.1080/1828051X.2021.1963862, 2021.
Lucy, M. C., Butler, S. T., and Garverick, H. A.: Endocrine and metabolic mechanisms linking postpartum glucose with early embryonic and foetal development in dairy cows, Animal, 8, 82–90, https://doi.org/10.1017/S1751731114000482, 2014.
Lucy, M. S.: The role of glucose in dairy cattle reproduction, WCDS Adv. Dairy Technol., 28, 161–173, 2016.
McDonald, T. L., Larson, M. A., Mack, D. R., and Weber, A.: Elevated extrahepatic expression and secretion of mammary-associated serum amyloid A 3 (M-SAA3) into colostrum, Vet. Immunol. Immunopathol., 83, 203–211, https://doi.org/10.1016/s0165-2427(01)00380-4, 2001.
Miglio, A., Moscati, L., Fruganti, G., Pela, M., Scoccia, E., Valiani, A., and Maresca, C.: Use of milk amyloid A in the diagnosis of subclinical mastitis in dairy ewes, J. Dairy Res., 80, 496–502, https://doi.org/10.1017/S0022029913000484, 2013.
Minuti, A., Jahan, N., Lopreiato, V., Piccioli-Cappelli, F., Bomba, L., Capomaccio, S., Loor, J. J., Ajmone-Marsan, P., and Trevisi, E.: Evaluation of circulating leukocyte transcriptome and its relationship with immune function and blood markers in dairy cows during the transition period, Funct. Integr. Genomics, 20, 293–305, https://doi.org/10.1007/s10142-019-00720-0, 2020.
Morimatsu, M., Watanabe, A., Yoshimatsu, K., Fujinaga, T., Okubo, M., and Naiki, M.: Elevation of bovine serum C-reactive protein and serum amyloid P component levels by lactation, J. Dairy Res., 58, 257–261, https://doi.org/10.1017/s0022029900029836, 1991.
Moyes, K. M.: Triennial Lactation Symposium: Nutrient partitioning during intramammary inflammation: A key to severity of mastitis and risk of subsequent diseases?, J. Anim. Sci., 93, 5586–5593, https://doi.org/10.2527/jas.2015-8945, 2015.
Murata, H.: Stress and acute phase protein response: an inconspicuous but essential linkage, Vet. J., 173, 473–474, https://doi.org/10.1016/j.tvjl.2006.05.008, 2007.
Murata, H., Shimada, N., and Yoshioka, M.: Current research on acute phase proteins in veterinary diagnosis: an overview, Vet. J., 168, 28–40, https://doi.org/10.1016/S1090-0233(03)00119-9, 2004.
O'Reilly, E. L. and Eckersall, P. D.: Acute phase proteins: a review of their function, behaviour and measurement in chickens, Worlds. Poult. Sci. J., 70, 27–44, https://doi.org/10.1017/S0043933914000038, 2014.
Okonkwo, J. C., Omeje, I. S., Okonkwo, I. F., and Umeghalu, I. C. E.: Effects of breed, sex and source within breed on the blood bilirubin, cholesterol and glucose concentrations of Nigerian goats, Pak. J. Nutr., 9, 120–124, 2010.
Orro, T., Jacobsen, S., LePage, J.-P., Niewold, T., Alasuutari, S., and Soveri, T.: Temporal changes in serum concentrations of acute phase proteins in newborn dairy calves, Vet. J., 176, 182–187, https://doi.org/10.1016/j.tvjl.2007.02.010, 2008.
Panagiotakos, D. B., Pitsavos, C., Manios, Y., Polychronopoulos, E., Chrysohoou, C. A., and Stefanadis, C.: Socio-economic status in relation to risk factors associated with cardiovascular disease, in healthy individuals from the ATTICA study, Eur. J. Cardiovasc. Prev. Rehabil., 12, 68–74, 2005.
Petersen, H. H., Nielsen, J. P., and Heegaard, P. M. H.: Application of acute phase protein measurements in veterinary clinical chemistry, Vet. Res., 35, 163–87, https://doi.org/10.1051/vetres:2004002, 2004.
Poitou, C., Divoux, A., Faty, A., Tordjman, J., Hugol, D., Aissat, A., Keophiphath, M., Henegar, C., Commans, S., and Clément, K.: Role of serum amyloid a in adipocyte-macrophage cross talk and adipocyte cholesterol efflux, J. Clin. Endocrinol. Metab., 94, 1810–1817, https://doi.org/10.1210/jc.2008-2040, 2009.
Pyörälä, S.: Hirvonen's thesis on acute phase response in dairy cattle, University of Helsinki, Helsinki, ISBN: 951-45-9106-2, https://helda.helsinki.fi/handle/1975/55 (last access: 10 August 2022), 2000.
Rahman, M. M., Lecchi, C., Fraquelli, C., Sartorelli, P., and Ceciliani, F.: Acute phase protein response in Alpine ibex with sarcoptic mange, Vet. Parasitol., 168, 293–198, https://doi.org/10.1016/j.vetpar.2009.12.001, 2010a.
Rahman, M. M., Lecchi, C., Avallone, G., Roccabianca, P., Sartorelli, P., and Ceciliani, F.: Lipopolysaccharide-binding protein: Local expression in bovine extrahepatic tissues, Vet. Immunol. Immunopathol., 137, 28–35, https://doi.org/10.1016/j.vetimm.2010.04.006, 2010b.
Reynolds, L. P., Biondini, M. E., Borowicz, P. P., Vonnahme, K. A., Caton, J. S., Grazul-Bilska, A. T., and Redmer, D. A.: Functional significance of developmental changes in placental microvascular architecture, Endothelium, 12, 11–19, https://doi.org/10.1080/10623320590933734, 2005.
Sacks, G. P., Studena, K., Sargent, K., and Redman, C. W.: Normal pregnancy and preeclampsia both produce inflammatory changes in peripheral blood leukocytes akin to those of sepsis, Am. J. Obstet. Gynecol., 179, 80–86, https://doi.org/10.1016/s0002-9378(98)70254-6, 1998.
Sandri, S., Urban Borbely, A., Fernandes, I., de Oliveira, E. M., Knebel, F. H., Ruano, R., Zugaib, M., Filippin-Monteiro, F., Bevilacqua, E., and Campa, A.: Serum amyloid A in the placenta and its role in trophoblast invasion, PLoS One, 9, e90881, https://doi.org/10.1371/journal.pone.0090881, 2014.
Saremi, B., Mielenz, M., Rahman, M. M., Hosseini, A., Kopp, C., Dänicke, S., Ceciliani, F., and Sauerwein, H.: Hepatic and extrahepatic expression of serum amyloid A3 during lactation in dairy cows, J. Dairy Sci., 96, 6944–6954, https://doi.org/10.3168/jds.2013-6495, 2013.
Satué, K., Calvo, A., Muñoz, A., Fazio, E., and Medica, P.: Interrelationship between reproductive hormones and acute phase proteins during estrous cycle and pregnancy in Spanish purebred broodmares, Vet. Anim. Sci., 14, 100212, https://doi.org/10.1016/j.vas.2021.100212, 2021.
Sauerwein, H., Blees, T., Zamarian, V., Catozzi, C., Müller, U., Sadri, H., Dänicke, S., Frahm, J., and Ceciliani, F.: Acute phase proteins and markers of oxidative status in water buffalos during the transition from late pregnancy to early lactation, Vet. Immunol. Immunopathol., 228, 110113, https://doi.org/10.1016/j.vetimm.2020.110113, 2020.
Schoonjans, K., Staels, B., and Auwerx, J.: Role of the peroxisome proliferator-activated receptor (PPAR) in mediating the effects of fibrates and fatty acids on gene expression, J. Lipid Res., 37 907–25, 1996.
Sharma, A., Kumar, P., Singh, M., and Vasishta, N. K.: Haemato-biochemical and endocrine profiling of north western Himalayan Gaddi sheep during various physiological/reproductive phases, Open Vet. J., 5, 103–107, 2015.
Shingfield, K. J., Bernard, L., Leroux, C., and Chilliard, Y.: Role of trans fatty acids in the nutritional regulation of mammary lipogenesis in ruminants, Animal, 4, 1140–1166, https://doi.org/10.1017/S1751731110000510, 2010.
Sordillo, L. M., Contreras, G. A., and Aitken, S. L.: Metabolic factors affecting the inflammatory response of periparturient dairy cows, Anim. Heal. Res. Rev., 10, 53–63, https://doi.org/10.1017/S1466252309990016, 2009.
Tharwat, M., Ali, A., and Al-Sobayil, F.: Hematological and biochemical profiles in goats during the transition period, Comp. Clin. Pathol., 24, 1–7, https://doi.org/10.1007/s00580-013-1842-1, 2013.
Tóthová, C., Nagy, O., and Kovác, G.: Changes in the concentrations of selected acute phase proteins and variables of energetic profile in dairy cows after parturition, J. Appl. Anim. Res., 42, 278–283, https://doi.org/10.1080/09712119.2013.842485, 2014a.
Tóthová, C., Nagy, O., and Kováč, G.: Relationship between some variables of protein profile and indicators of lipomobilization in dairy cows after calving, Arch. Anim. Breed., 57, 19, https://doi.org/10.7482/0003-9438-57-019, 2014b.
Ulutas, P. A., Musal, B., Kiral, F., and Bildik, A.: Acute phase protein levels in pregnancy and oestrus cycle in bitches, Res. Vet. Sci., 86, 373–376, https://doi.org/10.1016/j.rvsc.2008.09.001, 2009.
Vasto, S., Candore, G., Balistreri, C. R., Caruso, M., Colonna-Romano, G., Grimaldi, M. P., Listi, F., Nuzzo, D., Lio, D., and Caruso, C.: Inflammatory networks in ageing, age-related diseases and longevity, Mech. Ageing Dev., 128, 83–91, https://doi.org/10.1016/j.mad.2006.11.015, 2007.
Waziri, M. A., Abdullahi, Y., Ribadu, A. Y., and Sivachelvan, N.: Changes in the serum proteins, hematological and some serum biochemical profiles in the gestation period in the Sahel goats, Vet. Arh., 80, 215–224, 2010.
Werling, D., Sutter, F., Arnold, M., Kun, G., Tooten, P. C., Gruys, E., Kreuzer, M., and Langhans, W.: Characterisation of the acute phase response of heifers to a prolonged low dose infusion of lipopolysaccharide, Res. Vet. Sci., 61, 252–257, https://doi.org/10.1016/s0034-5288(96)90073-9, 1996.
Yamashita, K., Fujinaga, T., Okumura, M., Takiguchi, M., Tsunoda, N., and Mizuno, S.: Serum C-reactive protein (CRP) in horses: the effect of aging, sex, delivery and inflammations on its concentration, J. Vet. Med. Sci., 53, 1019–1024, https://doi.org/10.1292/jvms.53.1019, 1991.