the Creative Commons Attribution 4.0 License.
the Creative Commons Attribution 4.0 License.
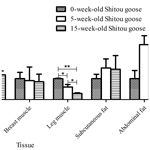
Polymorphism analysis and expression patterns of the IGF1 gene in the Shitou goose
Mao Guo
Jing Fu
Hongjia Ouyang
Yunbo Tian
Xu Shen
Yunmao Huang
Insulin-like growth factor 1 (IGF1) is one of the endocrine hormones that plays an important role in regulating growth and development of animals. In this study, polymorphism in the 5′UTR and 3′UTR coding region and of the IGF1 gene was detected by DNA sequencing technology, and the abundance of IGF1 mRNA in various tissues at three growth stages of the Shitou goose was determined by quantitative real-time polymerase chain reaction (qRT-PCR). Moreover, the differential expression of IGF1 in various tissues between the Shitou goose and Wuzong goose was revealed. Two single nucleotide polymorphisms (SNPs) were found in the exon3 region of IGF1 in the Shitou goose. IGF1 mRNA was extensively expressed in various tissues of Shitou geese with high abundant expression in the liver, breast muscle and leg muscle at three growth stages. IGF1 mRNA expression showed a trend of first increase and then decrease in the pituitary, liver, subcutaneous fat and abdominal fat tissues, but it decreased in the breast muscle and leg muscle of a Shitou goose with growing age. Expression of IGF1 in the liver, leg muscle and pituitary tissues of the Shitou goose was significantly higher than that of the Wuzong goose. This provides a foundation for further study of regulatory mechanisms of IGF1 in the growth and development of geese.
- Article
(1823 KB) - Full-text XML
- BibTeX
- EndNote
China is the largest goose-producing and goose-consuming country in the world. In 2018, 530 million commercial geese were for sale, and the number of geese in China accounted for more than 93.3 % in the world (Hou, 2019). Goose meat products are low in fat and cholesterol but high in protein. The protein content of goose meat reaches as high as 22.3 %, but its fat content is low at only about 11 %, of which unsaturated fatty acid content accounts for more than 99 % of the total (Mary, 1989). It is recognized as a healthy food and tremendously loved by consumers. Goose breeding is a profitable business in the contemporary the poultry industry. Production of poultry meat is closely related to its growth traits. Growth traits are important economic traits in poultry industry, which are controlled by genes and the environment (Goto et al., 2019). Therefore, studies on genes related to poultry growth and development represent a hot topic of interest.
Insulin-like growth factor 1 (IGF1) is one of the endocrine hormones that plays an important role in regulating growth, development, metabolism and reproduction of animals (Herbert et al., 2000). IGF1 was first discovered by Salmon and Daughaday in 1957 (Salmon and Daughaday, 1957). It is a single-chain polypeptide composed of 70 amino acids with a relative molecular weight of 7649D (Bortvedt and Lund, 2012). Studies have found that IGF1 can stimulate and promote the differentiation of muscle cells to participate in the genesis of skeletal muscle (Florini et al., 1991, 1996). Growth and development of animal muscle are closely related to temporal and spatial expression of IGFs (Tilley et al., 2007). Bhattacharya et al. (2015) found that IGF1 expression in muscle was highest on day 1 and day 28, whereas the lowest expression was on day 42 in chickens. Hu et al. (2015) studied the expression of the IGF1 gene in the pectoralis major muscle and lateral gastrocnemius muscle of a Gaoyou duck and a Jinding duck with different growth rates at the embryonic stage and 7 d after hatching; they found that the expression level of IGF1 in the pectoralis major muscle of a Gaoyou duck was significantly higher than that of a Jinding duck at 13 and 21 embryonic days and 7 d of age. IGF1 mRNA expression in chicken liver, which is the main organ for IGF1 mRNA expression (Peng et al., 1996), is positively correlated with body weight increase (Beccavin et al., 2001). Studies on the molecular mechanism of IGF1 are mainly focused on other animals, but there are few on geese.
The Shitou goose is the largest goose species originating from Anser cygnoides in the world, which is from Raoping County, Guangdong Province, China. The average weight of a male Shitou goose can be up to 10.39 kg (Lin et al., 2003), with excellent growth performance. The Wuzong goose is the smallest goose species originating from Anser cygnoides in the world, which is from Qingyuan City, Guangdong Province, China. The weight of a male Wuzong goose is only 3–3.5 kg (Gong, 2008), which is less than one-third of the Shitou goose, and its growth performance is very different. In addition, Tu et al. (2006) found that Shitou geese and Wuzong geese were clustered into one group through an unweighted pair-group method with arithmetic means (UPGMA) cluster analysis. It is suggested that the genetic background of Shitou geese and Wuzong geese is similar. It is an ideal model for studying the growth and development of Chinese local geese. In this study, mRNA expression patterns of IGF1 in the hypothalamus, pituitary, liver, breast muscle, leg muscle, subcutaneous fat and abdominal fat tissues of 0, 5 and 15-week-old Shitou geese were detected by qRT-PCR. Meanwhile, differences of IGF1 mRNA expression in these seven tissues were compared between a Shitou goose and Wuzong goose at 5 weeks of age, and polymorphism of IGF1 was screened and analyzed.
2.1 Ethics statement
Housing, management and care of the birds conformed to the guidelines of the Animal Care and Use Committee at Zhongkai University of Agriculture and Engineering (approval number 2020-012) and also in accordance with the Animal Protection Law of the People's Republic of China.
2.2 Experimental animals and sample collection
The test birds used in this experiment were large-sized Shitou geese and small-sized Wuzong geese from Qingyuan Jinyufeng Goose Industry Co., Ltd (Guangdong, China). All the birds used in this experiment were raised in the same batch; they were free to eat and drink and were supplemented with green grass as much as possible during feeding. Three male Shitou geese were selected and slaughtered at 0, 5 and 15 weeks of age, and three male Wuzong geese were selected and slaughtered at 5 weeks of age. After slaughtering, hypothalamus, pituitary, liver, breast muscle, leg muscle, subcutaneous fat and abdominal fat tissues were collected and quickly transferred to liquid nitrogen for RNA extraction and qRT-PCR. A total of 57 Shitou geese blood samples were collected and stored at −80∘ for DNA extraction.
2.3 DNA and RNA isolation and first-strand cDNA synthesis
DNA was isolated from goose blood using a Blood Genomic DNA Extraction Kit (Solarbio, China) according to the manufacturer's protocol and diluted to 100 ng µL−1. Total RNA was extracted from each tissue using TRIzol reagent (Invitrogen, Carlsbad, CA, USA) according to the manufacturer's protocol. Purified total RNA was dissolved in RNAase-free water and quantified by Nanodrop 2000 (Thermo, USA) at 260 nm. The RNA (3 µg) was synthesized to cDNA using a PrimeScript™ RT reagent kit with a gDNA Eraser reverse transcription kit (TaKaRa, Japan) according to the manufacturer's protocol and then stored at −20 ∘C for qRT-PCR analysis.
2.4 Design of primers, PCR and qRT-PCR
The IGF1 gene consists of 5′UTR, four exons and 3′UTR. The primers were designed from the available goose IGF1 sequence (GenBank accession no. NW_013185696.1) using Primer 5.0 software (Premier Biosoft International, Palo Alto, CA, USA). Moreover, primers for reference genes were also designed (Table 1).
The PCR reaction was set up with 30 µg of DNA template, 10 ng of each primer, 1.5 mM of MgCl2, 100 µM of each dNTP, 1× assay buffer and 0.25 U of Taq DNA polymerase (MBI Fermentas, Amherst NY, USA). PCR was performed with initial denaturation at 94 ∘C for 5 min, 33 cycles of denaturation at 94 ∘C for 30 s, annealing at a specific temperature (Table 1) and extension at 72 ∘C for 30 s with a final extension at 72 ∘C for 5 min.
The qRT-PCR analysis was performed in triplicate using an iTaqTM Universal SYBR® Green Supermix Kit (Bio-Rad, Hercules, CA, USA) on an ABI QuantStudio 5 Real-Time PCR System (ABI, CA, USA). The relative expression levels were determined using the 2−ΔΔCt method (Livak and Schmittgen, 2001) with β-actin as a control. The reaction conditions were as follows: 95 ∘C for 10 min, 40 cycles of 95 ∘C for 15 s and 56 ∘C for 60 s.
2.5 Polymorphism screening and genotyping
A total of 20 samples of Shitou geese DNA diluted to the same concentration were randomly selected and mixed to construct a DNA pool. PCR products from the DNA pool were sequenced to screen for gene polymorphism. Genotype mutations of each sample were analyzed by sequencing performed by Shanghai Shenggong Biological Co., Ltd. A total of 57 Shitou geese were used for polymorphism analysis.
2.6 Data analysis
Lasergene software was used to analyze the alleles. Genotype and allele frequencies of the Shitou goose were calculated and Hardy–Weinberg equilibrium (HWE) was calculated by the SHEsis program (Shi and He, 2005). In addition, population indexes such as effective allele numbers (Ne), polymorphic information content (PIC) and heterozygosity (He) were calculated following Nei's methods (Nei and Roychoudhury, 1974) performed by PopGene (version 1.3.1, AB, Canada). Significant differences between two samples and multi-groups were analyzed by one-way analysis of variance (ANOVA) and least square means for multiple comparisons using the SPSS 22.0 software.
3.1 Sequence variation and polymorphisms analysis
There is no variation except two SNPs in the exon3 region of IGF1, in which there are nucleotide substitutions at positions 40 740 and 40 825 (Fig. 1), named as g.40740G>C and g.40825G>T. Three genotypes of two SNPs detected by sequencing are shown in Fig. 2.
Results of genetic polymorphism analysis of two SNPs are shown in Table 2. The major allele of g.40740G>C locus was G, with a frequency of 74.56 %. The major genotype was GG, with a frequency of 54.39 %. The g.40740G>C locus was moderately polymorphic (0.25< PIC <0.5) in Hardy–Weinberg equilibrium (χ2>0.05). The major allele of g.40825G>T locus was G, with a frequency of 70.18 %. The major genotype was TG, with a frequency of 56.14 %. The g.40825G>T locus was moderately polymorphic (0.25< PIC <0.5) in Hardy–Weinberg disequilibrium (χ2<0.05).
3.2 Tissue expression of IGF1 in a 0-week-old Shitou goose
Taking the expression level of IGF1 in the hypothalamus of a 0-week-old Shitou goose as a reference, the relative expression level of IGF1 in seven different tissues of a 0-week-old Shitou goose is shown in Fig. 3. IGF1 mRNA was ubiquitously expressed in all examined tissues (hypothalamus, pituitary, liver, breast muscle, leg muscle, subcutaneous fat and abdominal fat) with different abundances. Expression of IGF1 in breast muscle was highest, which was significantly higher than that in the hypothalamus and subcutaneous fat (P<0.05), as well as the pituitary gland and abdominal fat (P<0.01). The second-highest expression of IGF1 was in liver and leg muscle, while the lowest was in the pituitary gland. It is suggested that IGF1 is possibly related to muscle growth and development of the Shitou goose at the time of hatching.
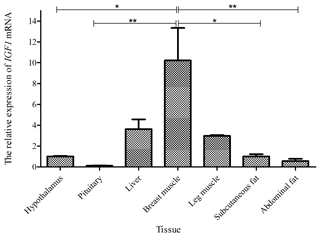
Figure 3The mRNA expression of IGF1 in different tissues of a 0-week-old Shitou goose. The * symbol represents a significant difference (P<0.05). The symbol represents a very significant difference (P<0.01). The same applies to Figs. 4 to 7. Values are shown as the mean ± SD (n=3); the same applies to Figs. 4 to 7.
3.3 Tissue expression of IGF1 in a 5-week-old Shitou goose
Taking the expression level of IGF1 in the hypothalamus of a 5-week-old Shitou goose as a reference, the relative expression level of IGF1 in seven different tissues of a 5-week-old Shitou goose is shown in Fig. 4. The expression level of IGF1 in the liver was highest, which was significantly higher than that in other tissues (P<0.01). The expression level of the IGF1 gene in breast muscle was significantly higher than that in the hypothalamus and pituitary tissue. Expression in leg muscle, subcutaneous fat and abdominal fat was also higher. It is suggested that IGF1 probably has an influence mainly on functions in the liver and the growth and development of muscle and adipose tissue at 5 weeks of age.
3.4 Tissue expression of IGF1 in a 15-week-old Shitou goose
Taking the expression level of IGF1 in the hypothalamus of a 15-week-old Shitou goose as a reference, the relative expression level of IGF1 in seven different tissues of a 15-week-old Shitou goose is shown in Fig. 5. The expression level of IGF1 in breast muscle was highest, which was significantly higher than that in other tissues except the liver. Expression of IGF1 in subcutaneous fat, abdominal fat, hypothalamus, leg muscle and pituitary tissue was low, and the difference was not significant between them.
3.5 Expression patterns of the IGF1 gene in the same tissue at different weeks of Shitou geese
Taking the expression level of IGF1 in a 0-week-old Shitou goose in different tissues as a reference, the relative expression level of IGF1 in the same tissue at different growth stages is shown in Fig. 6. Expression of IGF1 in pituitary, liver, subcutaneous fat and abdominal fat tissues of a Shitou goose showed a trend of first increase and then decrease with the increase in age. However, the expression of IGF1 in the hypothalamus showed a trend of first decrease and then increase with the increase in age. Expression of IGF1 in breast muscle and leg muscle decreased with the increase in age. It can be seen that the expression of IGF1 in different stages of the same tissue is quite different.
3.6 Differential expression analysis of IGF1 in different tissues of a 5-week-old Shitou goose and Wuzong geese
Taking the expression level of IGF1 in a 5-week-old Wuzong goose as a reference, the differential expression of IGF1 in different tissues of a 5-week-old Shitou goose and Wuzong goose is shown in Fig. 7. The expression level of IGF1 in the liver and leg muscle tissues of a 5-week-old Shitou goose was significantly higher than that of a Wuzong Goose (P<0.01), and the expression level of IGF1 in the pituitary tissue of a 5-week-old Shitou goose was significantly higher than that of a Wuzong goose (P<0.05). Expression of the IGF1 gene in the hypothalamus of a 5-week-old Shitou goose was significantly lower than that of a Wuzong goose (P<0.05).
IGF1 is an important growth regulator, which plays a vital role in animal growth and development through autocrine and paracrine functioning (Bondy and Cheng, 2004). Therefore, the study of IGF1 gene polymorphism and expression patterns is helpful to understand the regulatory mechanism of IGF1 in animal growth and development.
The regulation of IGF1 in animal growth and development has established a relationship between polymorphism and growth performance. Bian et al. (2008) identified three SNPs (c.−366A>C, c.528G>A and c.*1024C>T) that constructed haplotypes in the chicken IGF1 gene. Haplotypes based on three polymorphisms were associated with body weight traits. Sato et al. (2011) revealed that a single nucleotide polymorphism (g.570C>A) in IGF1 was significantly associated with breast muscle yields in the F2 population for which it was an intercross between a high-breast-muscle-yield male line and a low-breast-muscle-yield female line in chickens. In this study, two SNPs were found in the exon3 region of the IGF1 gene in Shitou geese. The PIC of two SNPs was moderate, and the G-40740C locus was in a Hardy–Weinberg equilibrium state. The proportion of homozygous CC (g.40740G>C) and TT (g.40825G>T) is very low, but the proportion of heterozygosity is about 50 %. The potential reason may be the Shitou goose undergoing artificial selection during evolution. It provided a foundation for revealing the relationship between goose growth performance and IGF1 gene polymorphism, and it also provided some reference for goose breeding.
In this study, it was found that IGF1 mRNA was expressed in the hypothalamus, pituitary gland, liver, breast muscle, leg muscle, subcutaneous fat and abdominal fat at three growth stages of 0, 5 and 15 weeks of age. Expression of IGF1 in the liver was high in all three growth stages. Similar results have also been reported in Jinghai yellow chickens (Abdalhag et al., 2016) and pigs (Peng et al., 1996), which indicates that the liver is the major endocrine source of IGFs (Zahran and Aboul-Soud, 2008; Georgiev, 2010). IGF1 is also a kind of high-performance muscle nutrition factor, which plays an important role in animal protein deposition and muscle cell proliferation. Its expression in muscle can significantly promote growth and development of muscle (Mitchell, 2002; Coleman, 1995). In the three growth stages of this study, expression of IGF1 was always higher in the breast muscle and leg muscle except for the leg muscle at 15 weeks of age, indicating that the expression of IGF1 may promote muscle growth and development in the Shitou goose.
With the increase in age, expression of IGF1 showed different patterns in different tissues. Expression of IGF1 in the pituitary, liver, subcutaneous fat and abdominal fat tissues of a Shitou goose showed a trend of first increase and then decrease, with the highest at 5 weeks of age. However, 5 weeks of age is the high-speed growth and development period of a Shitou goose (Lu et al., 2002). The results showed that the expression of IGF1 was higher in most tissues during the rapid growth and development period, indicating that IGF1 probably plays a role in both growth and development of geese. This is similar to other studies in that a highly variable relationship exists between circulating IGF1 and post-hatch growth in chickens (Vasilatos-Younken and Scanes, 1991), and a positive correlation (r=0.62) exists between circulating concentrations of IGF1 and body weight (Goddard et al., 1988). IGF1, the expression of which is increased during the differentiation of human preadipocytes, can also promote the proliferation and differentiation of preadipocytes (Kelly et al., 2010; Bouraoui et al., 2010; Susann et al., 2005), and both preadipocytes and adipocytes can secrete IGF1 (Hemkendreis, 2000). Therefore, the high expression of IGF1 in adipose tissue can promote the growth and development of adipose tissue. However, the expression of IGF1 in breast muscle and leg muscle decreased gradually with aging, which was similar to a study conducted by Peng et al. (1996), who reported a decrease in the expression of the IGF1 gene in pig skeletal muscle (Peng et al., 1996). At the same time, it may be related to the earlier development of breast and leg muscles. Yun et al. (2005) found that the breast muscle gains weight most rapidly in 1–3 weeks, which was more than 5 times the birth weight. Therefore, IGF1 expressed abundantly in early stages promotes the rapid growth of muscle.
There are great differences in growth traits between Shitou geese and Wuzong geese, which is an ideal model for studying bird growth and development. In this study, the expression of IGF1 in liver and leg muscle tissues of a Shitou goose was significantly higher than that of a Wuzong goose, and the expression level of the IGF1 gene in pituitary tissue of a Shitou goose was significantly higher than that of a Wuzong goose. Liver and muscle are the main organs for IGF1 secretion and function; IGF1 mRNA expression in chicken liver is positively correlated with body weight increase (Beccavin et al., 2001), and the expression of IGF1 in muscle can noticeably promote the growth and development of muscle (Mitchell et al., 2002; Coleman et al., 1995). Hence, it is indicated that IGF1 may play an important role in regulating the rapid growth of Shitou geese.
In this study, two SNPs were found in the exon3 region of the IGF1 gene in Shitou geese. IGF1 mRNA expression was higher in most tissues during the rapid growth and development period and was extensively expressed in various tissues of a Shitou goose with different abundances at all three growth stages. Expression of IGF1 in the liver, leg muscle and pituitary tissues of a Shitou goose was very significantly or significantly higher than that of a Wuzong goose, indicating that the IGF1 gene may play an important role in regulating the growth and development of geese.
The original data of the paper are available from the corresponding author upon request.
JT and YH designed the research. MG and XS performed the material preparation and experiments. XS, HO and YT performed the data collection and analysis. JT and JF drafted the paper. All authors read and approved the final paper.
The authors declare that they have no conflict of interest.
Publisher's note: Copernicus Publications remains neutral with regard to jurisdictional claims in published maps and institutional affiliations.
We thank Qingyuan Jinyufeng Goose Industry Co., Ltd. for providing experimental animals.
This work was funded by the Key-Area Research and Development Program of Guangdong Province (grant no. 2020B020222003), the National Natural Science Foundation of China (grant no. 31902164), the Major Fundamental Research Project of Educational Department of Guangdong Province (grant no. 2018KZDXM039), the Provincial Undergraduate Training Program for Innovation and Entrepreneurship (grant no. 202011347044), and the characteristic innovation projects of ordinary colleges and universities in Guangdong Province (grant no. 2018KTSCX102).
This paper was edited by Steffen Maak and reviewed by two anonymous referees.
Abdalhag, M. A., Li, T., Duan, L., Zhang, T., Zhang, G., and Wang, J.: Association analysis of IGF-I gene expression with growth and reproductive traits in Jinghai yellow chickens, Genet. Mol. Res., 15, 1–11, https://doi.org/10.4238/gmr15049205, 2016.
Beccavin, C., Chevalier, B., Cogburn, L. A., Simon, J., and Duclos, M. J.: Insulin-like growth factors and body growth in chickens divergently selected for high or low growth rate, J. Endocrinol., 168, 297–306, https://doi.org/10.1677/joe.0.1680297, 2001.
Bhattacharya, T. K., Chatterjee, R. N., Dushyanth, K., Paswan, C., Shukla, R., and Shanmugam, M.: Polymorphism and expression of insulin-like growth factor 1 (IGF1) gene and its association with growth traits in chicken, Brit. Poultry. Sci., 56, 398–407, https://doi.org/10.1080/00071668.2015.1041098, 2015.
Bian, L. H., Wang, S. Z., Wang, Q. G., Zhang, S., Wang, Y. X., and Li, H.: Variation at the insulin-like growth factor 1 gene and its association with body weight traits in the chicken, J. Anim. Breed. Genet., 125, 265–270, https://doi.org/10.1111/j.1439-0388.2008.00739.x, 2008.
Bondy, C. A. and Cheng, C. M.: Signaling by insulin-like growth factor 1 in brain, Eur. J. Clin. Pharmacol., 490, 25–31, https://doi.org/10.1016/j.ejphar.2004.02.042, 2004.
Bortvedt, S. F. and Lund, P. K.: Insulin-like growth factor 1: common mediator of multiple enterotrophic hormones and growth factors, Curr. Opin. Gastroen., 28, 89–98, https://doi.org/10.1097/MOG.0b013e32835004c6, 2012.
Bouraoui, L., Capilla, E., Gutiérrez, J., and Navarro, I.: Insulin and insulin-like growth factor I signaling pathways in rainbow trout (Oncorhynchus mykiss) during adipogenesis and their implication in glucose uptake, Am. J. Physiol. Regul. Integr. Comp. Physiol., 299, R33–R41, https://doi.org/10.1152/ajpregu.00457.2009, 2010.
Coleman, M. E., DeMayo, F., Yin, K. C., Lee, H. M., Geske, R., Montgomery, C., and Schwartz, R. J.: Myogenic vector expression of insulin-like growth factor I stimulates muscle cell differentiation and myofiber hypertrophy in transgenic mice, J. Biol. Chem., 270, 12109–121016, https://doi.org/10.1074/jbc.270.20.12109, 1995.
Florini, J. R., Magri, K. A., Ewton, D. Z., James, P. L., Grindstaff, K., and Rotwein, P. S.: “Spontaneous” differentiation of skeletal myoblasts is dependent upon autocrine secretion of insulin-like growth factor-II, J. Biol. Chem., 266, 15917–15923, https://doi.org/10.1016/0005-2736(91)90052-A, 1991.
Florini, J. R., Ewton, D. Z., and Coolican, S. A.: Growth hormone and the insulin-like growth factor system in myogenesis, Endocr. Rev., 17, 481–517, https://doi.org/10.1210/edrv-17-5-481, 1996.
Georgiev, I. P.: Physiological characteristics of the somatotropin-insulin-like growth factors axis in calves during the foetal and postnatal development, Bulg. J. Vet. Med., 13, 140–147, 2010.
Goddard, C., Wilkie, R. S., and Dunn, I. C.: The relationship between insulin-like growth factor-1, growth hormone, thyroid hormones and insulin in chickens selected for growth, Domest. Anim. Endocrin., 5, 165–176, https://doi.org/10.1016/0739-7240(88)90017-3, 1988.
Gong, G.: Small-size local goose with Wuzong goose., Breeding World, 4, 41–41, 2008.
Goto, T., Ishikawa, A., Nishibori, M., and Tsudzuki, M.: A longitudinal quantitative trait locus mapping of chicken growth traits, Mol. Genet. Genomics, 294, 243–252, https://doi.org/10.1007/s00438-018-1501-y, 2019.
Hemkendreis, B.: IGF-I- and IGFBP-3-Expression in Cultured Human Preadipocytes and Adipocytes, Horm. Metab. Res, 32, 555–559, https://doi.org/10.1055/s-2007-978685, 2000.
Herbert, Y. and Thomas, R.: Role of the Insulin-Like Growth Factor Family in Cancer Development and Progression, J. Natl. Cancer Inst., 92, 1472–1489, https://doi.org/10.1093/jnci/92.18.1472, 2000.
Hou, S. S.: Development status, future development trend and suggestions of waterfowl industry in 2018 [J], Chinese Journal of Animal Husbandry, 55, 124–128, 2019.
Hu, Y., Liu, H., Shan, Y., Ji, G., Xu, W., Sh, J., and Li, H.: The relative expression levels of insulin-like growth factor 1 and myostatin mRNA in the asynchronous development of skeletal muscle in ducks during early development, Gene, 567, 235–243, https://doi.org/10.1016/j.gene.2015.05.001, 2015.
Kelly, C. D., Maile, L. A., Tsiaras, W. G., Tamara, T., Kirkland, J. L., and Boney, C.: M.IGF-I activation of the AKT pathway is impaired in visceral but not subcutaneous preadipocytes from obese subjects, Endocrinology, 151, 3752–3763, https://doi.org/10.1210/en.2010-0043, 2010.
Lin, Z. and Lin, Q.: Investigation on the resources of Shitou geese, Guide Chinese Poultry, 20, 36–37, 2003.
Livak, K. J. and Schmittgen, T. D.: Analysis of Relative Gene Expression Data Using Real-Time Quantitative PCR and the 2 (−ΔΔC(T)) Method, Methods, 25, 402–408, https://doi.org/10.1006/meth.2001.1262, 2001.
Lu, M., Ye, R., and Yang, X.: Analysis of Growth Curves of Lionhead Geese under Different Feeding Manners, China Poultry, 24, 14–15, 2002.
Mary, H. S.: Nutrition of domestic geese, P. Nutr. Soc., 48, 103–111, https://doi.org/10.1079/PNS19890014, 1989.
Mitchell, P. J., Johnson, S. E., and Hannon, K.: Insulin-like growth factor I stimulates myoblast expansion and myofiber development in the limb, Dev. Dynam., 223, 12–23, https://doi.org/10.1002/dvdy.1227, 2002.
Nei, M. A. and Roychoudhury, A. K.: Sampling variances of heterozygosity and genetic distance, Genetics, 76, 379–390, 1974.
Peng, M., Pelletier, G., Palin, M. F., Veronnean, S., LeBel, D., and Abribat, T.: Ontogeny of IGFs and IGFBPs mRNA levels and tissue concentrations in liver, kidney and skeletal muscle of pig, Growth. Dev. Aging., 60, 171–187, https://doi.org/10.1016/S0197-4572(96)80218-7, 1996.
Salmon, W. D. and Daughaday, W. H.: A hormonally controlled serum factor which stimulates sulfate incorporation by cartilage in vitro, J. Lab. Clin. Med., 49, 825–836, https://doi.org/10.1007/BF02599443, 1957.
Sato, S., Ohtake, T., Uemoto, Y., Okumura, Y., and Kobayashi, E.: Polymorphism of insulin-like growth factor 1 gene is associated with breast muscle yields in chickens, Anim. Sci. J., 83, 1–6, https://doi.org/10.1111/j.1740-0929.2011.00917.x, 2011.
Shi, Y. Y. and He, L.: SHEsis, a powerful software platform for analyses of linkage disequilibrium, haplotype construction, and genetic association at polymorphism loci, Cell Res., 15, 97–98, https://doi.org/10.1038/sj.cr.7290272, 2005.
Susann, B., Jürgen, K., and Kiess, W.: Insulin-like growth factor I, growth hormone and insulin in white adipose tissue, Best. Pract. Res. Cl. En. Met., 19, 577–587, https://doi.org/10.1016/j.beem.2005.07.011, 2005.
Tilley, R. E., Mcneil, C. J., Ashworth, C. J., Page, K. R., and Mcardle, H. J.: Altered muscle development and expression of the insulin-like growth factor system in growth retarded fetal pigs, Domest. Anim. Endocrin., 32, 167–177, https://doi.org/10.1016/j.domaniend.2006.02.003, 2007.
Tu, Y., Chen, K., Tang, Q., Wang, J., Gao, Y., Gu, R., and Ge, Q.: Genetic diversity of 13 indigenous grey goose breeds in China based on microsatellite markers, Biodiversity Science, 14, 152–158, 2006.
Vasilatos-Younken, R. and Scanes, C. G.: Growth hormone and insulin-like growth factors in poultry growth: required, optimal, or ineffective, Poult. Sci., 70, 1764–1780, https://doi.org/10.3382/ps.0701764, 1991.
Yun, J. S., Seo, D. S., Kim, W. K., and Ko, Y.: Expression and relationship of the insulin-like growth factor system with posthatch growth in the korean native ogol chicken, Poult. Sci., 84, 83–90, https://doi.org/10.1093/ps/84.1.83, 2005.
Zahran, M. M. and Aboul-Soud, M. A.: Isolation and sequencing of insulin-like growth factor 1 (IGF-1) from Egyptian buffalo via RT-PCR, Arab. J. Biotech., 11, 19–28, 2008.