the Creative Commons Attribution 4.0 License.
the Creative Commons Attribution 4.0 License.
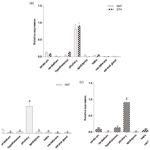
Expression analysis of DIO2, EYA3, KISS1 and GPR54 genes in year-round estrous and seasonally estrous rams
Qing Xia
Ran Di
Xiao-Yun He
Cai-Hong Wei
Ming-Xing Chu
The expression characteristics of the hypothalamic–pituitary–gonadal (HPG) axis-related candidate genes, DIO2, EYA3, KISS1 and GPR54, were analyzed in year-round estrous rams (small-tail Han sheep, STH) and seasonally estrous rams (Sunite sheep, SNT) using qPCR. The results were as follows: DIO2 was mainly expressed in pituitary, and KISS1 was specifically expressed in hypothalamus in the two groups. However, EYA3 and GPR54 were widely expressed in the cerebrum, cerebellum, hypothalamus, pituitary, testis, epididymis, vas deferens and adrenal gland tissues in both breeds, with significant differences in the cerebellum, hypothalamus, pituitary, testis and vas deferens tissues. We speculated that DIO2 and KISS1 may have positive roles in different regions in ram year-round estrus. Moreover, the expression patterns of EYA3 and GPR54 suggested that they may regulate the estrous mode of ram via testis and vas deferens. This is the first study to systematically analyze the expression patterns of HPG axis-related genes in rams.
- Article
(1650 KB) - Full-text XML
- BibTeX
- EndNote
Seasonal estrus is an important physiological behavior that animals have evolved to adapt to the environment, which is regulated by photoperiods (Dupré et al., 2010; Dardente et al., 2019). The photoperiodic mechanism in vertebrates is known to involve seasonal regulation of thyroid hormones, mediated in mammals via specialized cells (thyrotrophs) in the pars tuberalis (PT). Normally, the female estrous cycle is initiated by the melatonin from the hypothalamus, which functions in the pituitary and regulates the expression of photoperiodically induced genes (eyes absent 3 (EYA3), thyrotrophin β subunit (TSHβ) and chromogranin (CGA)). Then it induces a neuroendocrine cascade along the hypothalamic–pituitary–gonadal (HPG) axis in turn (Dupré et al., 2010; Tavolaro et al., 2014).
To date, some major genes affecting seasonal estrus in sheep have already been identified, such as period (PER), cryptochrome (CRY), BMAL1 and CLOCK, which all belong to the circadian rhythm genes. The expression levels of these genes are closely related to cycles in behavior and physiology (Marcheva et al., 2010; Janich et al., 2011; Nam et al., 2015).
Research has also found that HPG axis-related genes, type 2 deiodinase (DIO2), eyes absent 3 (EYA3), G protein-coupled receptor 54 (GPR54) and kisspeptin-1 (KISS1), are involved in regulating the mechanism of seasonal estrus in mammals (Dupré et al., 2010; Herbison et al., 2010; Tariq et al., 2013; Liu et al., 2017). The variations of DIO2/3 expression patterns in hypothalamic ependymal cells are regulated by melatonin secretion, which is associated with inactivation of THs and related to gonadal function (Lincoln, 1999; Ikegami and Yoshimura, 2012). EYA3, as the strongest long photoperiod-induced gene in sheep, is activated by a long photoperiod, revealing a common photoperiodic molecular response in birds and mammals (Dupré et al., 2010). GPR54 has been identified as the receptor of KISS1 (Muir et al., 2001; Ohtaki et al., 2001); it is vital for puberty onset and male fertility due to its central regulatory roles (Han et al., 2020). The loss-of-function mutations in GPR54 for humans and mice lead to the symptoms of idiopathic hypogonadotropic hypogonadism with the phenomenon of retarded sexual development and failure to reach puberty (Funes et al., 2003; Seminara et al., 2003; Roux et al., 2003). KISS1 neurons in the arcuate nucleus may regulate the negative feedback effect of gonadal steroids on GnRH and gonadotropin secretion in both sexes (Popa et al., 2008). Kisspeptins are potent secretagogues for GnRH, and the KISS1 gene is a target for regulation by gonadal steroids (e.g., estradiol and testosterone), metabolic factors (e.g., leptin), photoperiods, and seasons (Li et al., 2008). With an understanding of the associations of the four genes with seasonal estrus in female animals, there was a desire to gain further knowledge of how these genes could affect male reproduction.
Sheep (Ovis aries) are a typical seasonally estrous species (Tang et al., 2018). Small-tail Han sheep (STH) and Sunite sheep (SNT) are two native Chinese sheep breeds. In addition, STH has comparatively excellent characters, such as crude feed tolerance, rapid growth and good meat quality. Therefore, STH sheep provide an ideal model breed to explore the molecular genetic mechanisms related to year-round estrus in certain breeds (Miao et al., 2016). In contrast, Sunite sheep develop gonads and display seasonal reproductive behavior during specific times of the year (La et al., 2020). Therefore, the molecular mechanism of seasonal reproduction of sheep can be better studied by using Sunite sheep as a model. Results of all these previous studies indicated the DIO2, EYA3, KISS1 and GPR54 genes have important functions in female estrus. However, there have been few studies on the expression analyses in rams. Whether the four genes affect rams estrus remains to be elucidated. Therefore, in this study, we compared the tissue expression profiles and mRNA expression levels of the four genes in eight reproduction-related tissues between STH and SNT rams. Our study paves the way for an in-depth study of the seasonally estrous mode of rams.
2.1 Ethics statement and sample collection
All animals used in this study were approved by the Science Research Department (in charge of animal welfare issues) of the Institute of Animal Science, Chinese Academy of Agricultural Sciences (IAS-CAAS; Beijing, PR China). In addition, there was ethics approval by the animal ethics committee of IAS-CAAS (no. IAS2020-82, 28 July 2020).
Three STH rams were supplied by the Yuncheng Breeding Sheep Farm (Shandong province, China), and three SNT rams were from Urad Middle Banner, Inner Mongolia of China. All rams were healthy, approximately 2.5 years old and were kept in a sheltered outdoor paddock. All animals were euthanized (intravenous pentobarbital at 100 mg per kilogram), and eight tissues (cerebrum, cerebellum, hypothalamus, pituitary, epididymis, testis, vas deferens and adrenal gland) were collected from each animal. All tissues were frozen in liquid nitrogen immediately and then stored at −80 ∘C to be used for RNA extraction.
2.2 Total RNA extraction and cDNA synthesis
Total RNA was extracted from the eight collected tissues using a total RNA extraction kit for animal tissue (Tiangen, Beijing, China). Trizol (Invitrogen Inc., Carlsbad, CA, USA) was used to dissolve the tissues. The quantity and quality of total RNA were monitored using 1.5 % agarose gel electrophoresis (U = 150 V; 10 min) and ultraviolet spectrophotometry (UV-1201, Shimadzu, Kyoto, Japan), respectively. Then, the RNAs were stored at −80 ∘C until use.
The first strand of cDNA was prepared using a PrimeScript™ RT Reagent Kit according to the manufacturer's instructions (TaKaRa Bio Inc., Dalian, China). The PCR thermocycler program was as follows: 37 ∘C for 15 min, followed by 85 ∘C for 5 s. The reaction mixture contained 1.0 µL of PrimeScript RT Enzyme, 1.0 µL of random 6-mers, 4.0 µL of 5 × PrimeScript Buffer (for real time), 1.0 µL of total RNA and 13 µL of RNase-free ddH2O (total volume, 20 µL). Prior to storage at −80 ∘C, the standard working concentration of cDNA was 200 ng/µL. The quality of cDNA was evaluated by housekeeping gene (GAPDH) amplification, and cDNA was stored at −20 ∘C until use.
2.3 Primer design
Primers were designed with Premier 3.0 (version 4.1.0) (https://bioinfo.ut.ee/primer3-0.4.0/, last access: 15 October 2019). A total of five pairs of primers were designed to amplify different fragments of the ovine DIO2 (GenBank: XM_027972090.1), EYA3 (GenBank: NM_001161733.1), GPR54 (GenBank: NM_001318077.1), KISS1 (GenBank: NM_001306104.1) and GAPDH (GenBank: NM_001190390.1) genes, based on their assembled sequences in GenBank. All primers were synthesized by Beijing Tianyi Biotechnology Co., Ltd. (Beijing, China). The housekeeping gene (GAPDH) was used as an internal control to normalize the threshold cycle (Ct) values. Primer details are given in Table 1.
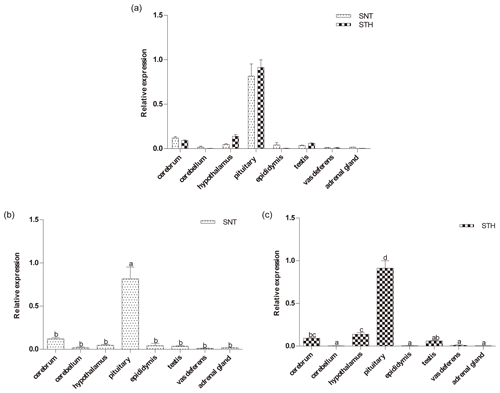
Figure 1The expression of DIO2 in eight tissues of small-tail Han sheep (STH) and Sunite sheep (SNT): (a) the comparison of the expression between SNT and STH, (b) the expression in SNT and (c) the expression in STH. Means with different superscripts are significantly different (P<0.05). The significant results with a p value lower than 0.05 are given one asterisk (∗) and lower than 0.01 are given two asterisks (), respectively.
2.4 qPCR
Real-time polymerase chain reaction (qPCR) amplification was performed in 20 µL of reaction mixture that contained 10 µL of SYBR Premix EX Taq II (TaKaRa Bio Inc., Dalian, China), 0.4 µL of each forward and reverse primer, 6.4 µL of RNase-Free ddH2O, and 2 µL of cDNA. PCR amplification was performed in triplicate wells using the following conditions: initial denaturation at 95 ∘C for 5 min, followed by 40 cycles of 95 ∘C for 10 s and 60 ∘C for 30 s. The dissociation curve was analyzed after amplification. A melting temperature (Tm) peak at 85 ∘C ± 0.8 on the dissociation curve was used to determine the specificity of PCR amplification.
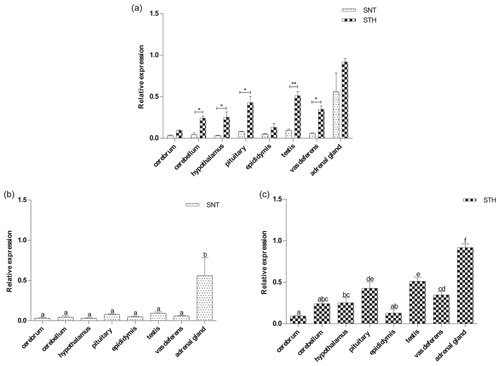
Figure 2The expression of EYA3 in eight tissues of small-tail Han sheep (STH) and Sunite sheep (SNT): (a) the comparison of the expression between SNT and STH, (b) the expression in SNT and (c) the expression in STH. Means with different superscripts are significantly different (P<0.05). The significant results with a p value lower than 0.05 are given one asterisk (∗) and lower than 0.01 are given two asterisks (), respectively.
2.5 Statistical analysis
The relative gene expression levels were calculated by the 2 method (Livak and Schmittgen, 2001; Livak, 2008). Statistical analyses were carried out using SPSS 19.0 software (IBM, Armonk, NY, USA). The levels of gene expression were analyzed for significant differences by one-way analysis of variance (ANOVA) followed by Fisher's least significant difference test as a multiple comparison test (Meier, 2006). All experimental data are presented as mean ± standard error of the mean (SEM).
3.1 Expression level of DIO2
As shown in Fig. 1, the expression level of DIO2 was similar in SNT and STH, with the highest level being in pituitary, followed by hypothalamus and cerebrum, with no significant difference between the two sheep breeds (Fig. 1a). However, DIO2 expression in pituitary was significantly higher than in the other seven tissues both in SNT and STH (P<0.05), and the expression level in hypothalamus was significantly higher than that in cerebellum, epididymis, testis, vas deferens and adrenal gland in STH (P<0.05) (Fig. 1b, c).
3.2 The expression level of EYA3
The results of the EYA3 expression analysis are shown in Fig. 2. EYA3 was widely expressed in the eight tissues in the two breeds. And EYA3 was expressed significantly higher in STH than SNT except for cerebrum and adrenal gland (P<0.05, P<0.01) (Fig. 2a). Additionally, EYA3 expression was significant higher in adrenal gland than that in other tissues both in SNT and STH (P<0.05) (Fig. 2b, c). Its expression in testis was significantly higher than other six tissues, with pituitary significantly higher than those of cerebrum, cerebellum, hypothalamus and epididymis in STH (P<0.05) (Fig. 2c). The expression of EYA3 in cerebellum, hypothalamus and epididymis had no significant difference in STH (Fig. 2c).
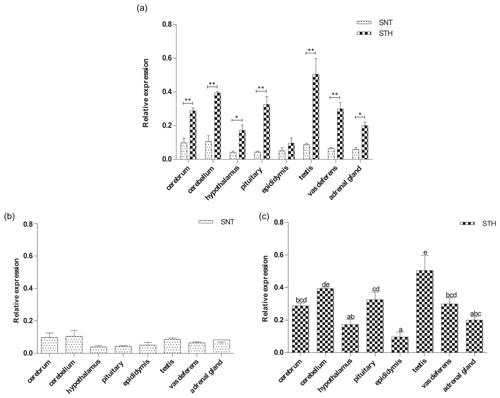
Figure 3The expression of GPR54 in eight tissues of small-tail Han sheep (STH) and Sunite sheep (SNT): (a) the comparison of the expression between SNT and STH, (b) the expression in SNT and (c) the expression in STH. Means with different superscripts are significantly different (P<0.05). The significant results with a p value lower than 0.05 are given one asterisk (∗) and lower than 0.01 are given two asterisks (), respectively.
3.3 The expression level of GPR54
Figure 3 clearly shows that GPR54 was widely expressed in all tissues in the two breeds, with a higher level in STH than SNT. The expression levels of GPR54 were reached at significant difference in hypothalamus and adrenal gland (P<0.05), with extremely significant difference in cerebrum, cerebellum, pituitary, testis and vas deferens between the two breeds (P<0.01) (Fig. 3a). There was no significant difference in SNT (Fig. 3b). And it was the highest in testis and the lowest in epididymis in STH (P<0.05) (Fig. 3c).
3.4 The expression level of KISS1
The results are shown in Fig. 4a. KISS1 was specifically expressed in hypothalamus, and the expression of KISS1 in hypothalamus is significantly higher in STH than SNT (P<0.01). The expression level of KISS1 was significantly higher in hypothalamus than that in pituitary, vas deferens and adrenal gland (P<0.05) in SNT (Fig. 4b); it was significantly higher in hypothalamus than other tissues in STH (P<0.05) (Fig. 4c).
4.1 DIO2 expression in seasonally estrous and year-round estrous sheep
DIO2 is known to be crucial for the seasonal estrous mode of female animals. It plays an important role in thyroid hormone metabolism and regulation (Park et al., 2018). DIO2 converts inactive (thyroxine, T4) to “active” thyroid hormone (3,5,3′-triiodothyronine, T3) and plays a significant role as a determinant of the final concentration of T3 (Dunn et al., 2017; Lomet et al., 2018; Dardente et al., 2019). Previous reports had found that DIO2 was upregulated in breeding compared to nonbreeding testes in male lizards, while DIO3 was upregulated in breeding ovaries in female lizards (Kang et al., 2020). Trivedi et al. (2019) found that DIO2 and EYA3 were highly expressed, and DIO3 and GnIH were lowly expressed in long photoperiods, concomitant with testis recrudescence in male migratory red-headed buntings. Therefore, the DIO2 gene has a certain impact on male reproduction. To our knowledge, no research on the expression of DIO2 in rams has ever been reported. In the present study, the highest expression of DIO2 was detected mainly in pituitary in STH and SNT rams. It further confirmed that DIO2 is associated with the pituitary function.
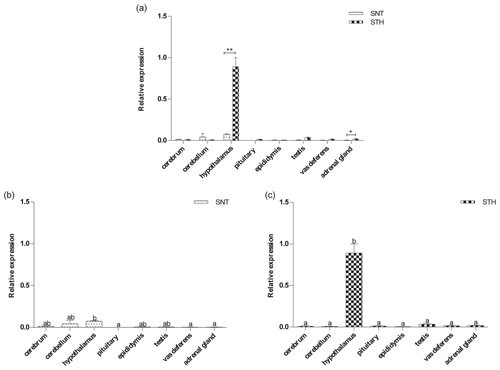
Figure 4The expression of KISS1 in eight tissues of small-tail Han sheep (STH) and Sunite sheep (SNT): (a) the comparison of the expression between SNT and STH, (b) the expression in SNT and (c) the expression in STH. Means with different superscripts are significantly different (P<0.05). The significant results with a p value lower than 0.05 are given one asterisk (∗) and lower than 0.01 are given two asterisks (), respectively.
Studies had found that DIO2 is widely expressed in the cerebrum, cerebellum, thyroid and testis in Brandt's vole and found that the core function of the DIO2 gene should be restricted in response to the photoperiod rather than factors directly regulating gonadal development (Liu et al., 2017). In addition, some researchers had found that DIO2 was expressed in the testes in rat and found DIO2 expression in adult rat testis was consistent with the participation of thyroid hormone in testicular function (Romano et al., 2017). In rodents, DIO2 was highly expressed in the hypothalamus (Tavolaro et al., 2014). In this research, DIO2 was shown to be highly expressed in the pituitary and widely expressed in the eight tissues. The result is similar to the study on Jining Grey goat and Liaoning cashmere goat, in which it was found that DIO2 was expressed in multiple tissues such as the cerebrum, cerebellum, hypothalamus, pituitary, ovary and uterus, and it was highly expressed in the pituitary and uterus (Huang et al., 2016). These indicated that its function is diverse and that it plays a critical role in the pituitary for ram seasonal estrus.
The expression of DIO2 in the hypothalamus, pituitary and testis is higher in STH than in SNT. This observation was different from previous studies comparing seasonally estrous and year-round estrous sheep (An et al., 2019a, b), in which ewes with seasonal estrus were reported to have a higher expression of DIO2 in the gonad tissues, which implies that rams may have a different regulation mechanism in estrus compared to ewes. Considering the function of DIO2 in conversion of thyroid hormone activity, it seems plausible that DIO2 may have a certain positive effect on ram year-round estrus. Of course, further studies should be performed to deeply investigate the relationship between DIO2 and ram estrus.
4.2 EYA3 expression in seasonally estrous and year-round estrous sheep
EYA genes had been identified as homologues of Drosophila eyes absent (EYA) genes. The eyes absent family has a protein phosphatase function, and its enzymatic activity is required for regulating genes encoding growth control and signaling molecules, modulating precursor cell proliferation (Li et al., 2003). The highly conserved vertebrate homologues Six1–6 (Oliver et al., 1996), Eya1-4 (Xu et al., 1997) and Dach1-2/Ski/Sno (Hammond et al., 1998) are co-expressed in multiple organs, including eye, inner ear, pituitary gland, muscle and kidney.
EYA3 can be expressed in thyroid-stimulating cells in the PT region, and it activates or inhibits the hypothalamic–pituitary–gonadal axis through the TSHβ-DIO2-TH pathway to achieve the regulation of seasonal estrus in sheep (Yoshimura, 2013; Dardente et al., 2014). Previous research had revealed that EYA3 plays an important role in regulating TSHβ in male CBA/N mice. EYA3 and its partner SIX1 synergistically activate TSHβ expression, and this activation is further enhanced by TEF and HLF (Masumoto et al., 2010). Likewise, long photoperiods caused a significant increase in EYA3, CGA, TSHβ and DIO2, followed by changes in testis size and plasma level of testosterone in male red-headed buntings (Park et al., 2018). Thus, EYA3 is an important candidate gene in male seasonal estrus.
Previous studies had shown that EYA3 as a photoperiod-induced gene is highly expressed in pituitary in female sheep, mice and birds (Dupré et al., 2010; Mishra et al., 2017; Masumoto et al., 2010). In this study, EYA3 was expressed in all eight tissues in rams, which implies that it plays a role in promoting the differentiation of many tissues. This is in agreement with EYA3 being widely expressed in the pituitary, pineal gland, cerebrum, cerebellum, hypothalamus, fallopian tube, ovary, adrenal gland and kidney in seasonal estrous and year-round estrous ewes (Xia et al., 2018). The highest expression of EYA3 in testis in STH ram indicated that EYA3 is associated with the testis function.
In addition, we compared the expression level of EYA3 in the selected eight tissues between two sheep breeds. We found the expression levels of EYA3 were significantly higher in cerebellum, hypothalamus, pituitary, testis and vas deferens of STH rams compared with SNT rams. Given the expression of EYA3 gene in the pituitary in ewes (Xu et al., 1997; Dupréet al., 2010), we speculated that its regulatory mechanism was similar in rams. EYA3 may have a certain positive effect on ram estrus.
4.3 KISS1 and GPR54 expression in seasonally estrous and year-round estrous sheep
Besides DIO2 and EYA3, the molecular mechanism of seasonal estrus involves the downstream signaling factor, including KISS1 and GPR54. They can regulate the release of hypothalamic gonadotropin-releasing hormone (GnRH), which in turn affects the secretion of follicle-stimulating hormone (FSH) and luteinizing hormone (LH), and are closely related to the onset of puberty and may be key genes for mammalian sexual maturity (Novaira et al., 2009; Papaoiconomou et al., 2011). KISS1 and GPR54 expression in mouse, rat, rhesus monkey and human testes indicates that this system has an autocrine or paracrine effect in the testis (Ohtaki et al., 2001; Terao et al., 2004; Tariq et al., 2013). In addition, they are contributing to stimulate testosterone secretion, testis maturation, gametogenesis and spermatozoid maturation in epididymis through FSH and LH (Scarlet et al., 2017; Feng et al., 2019). Smith et al. (2005a, b) found that KISS1 mediates testosterone and estrogen feedbacks on GnRH neurons and differentially responds to sex steroids. Exogenous administration of kisspeptins to sexually inactive male Syrian hamsters reverses the inhibitory effect of nonbreeding season by re-activating the HPG axis (Revel et al., 2006). Therefore, KISS1 and GPR54 have a certain impact on male seasonal estrus. But no evidence of the effect of KISS1 and GPR54 on seasonal estrus in rams has been reported so far. Therefore, KISS1 and GPR54 were selected for investigation of the seasonal estrus of rams in this study.
KISS1 was expressed in cerebrum and placenta in humans (Muir et al., 2001). Further studies revealed that KISS1 was expressed with high level in the hypothalamus in pigs (Tomikawa et al., 2010). GPR54 gene was widely expressed in many tissues in pigs, mice and Siberian hamsters (Li et al., 2008; Shahed et al., 2009; Herbison et al., 2010). KISS1 and GPR54 have also been implicated in regulating the estrus cycle of seasonal breeders and in the control of lactational amenorrhea (Colledge, 2008). Our results demonstrated that KISS1 was specifically expressed in hypothalamus both in SNT and STH. This is consistent with previous studies about seasonal estrous and year-round estrous goats (Huang et al., 2015) and ewes (An et al., 2019a). In addition, GPR54 was widely expressed in all selected tissues and highly expressed in the testis, cerebellum, pituitary and vas deferens, which implied that GPR54 may be positively correlated with the seasonal estrus of rams.
Numerous studies revealed that KISS1 and GPR54 had effects on testis in goats, mice, monkeys and humans (Terao et al., 2004; Tariq et al., 2013; Samir et al., 2015; Mei et al., 2013). In the present study, we compared the expression level of KISS1 and GPR54 in the testis, epididymis, and vas deferens between two sheep breeds. We found the expression levels of GPR54 in the testis and vas deferens of STH are significantly higher than in SNT rams. The KISS1 expression had no significant difference in testis between STH and SNT, while it was significantly higher in STH than in SNT in hypothalamus. Considering the core function of the hypothalamus in seasonal estrus, we speculated that KISS1 may play a key role in year-round estrus in hypothalamus of rams, similar to their reported functions in other mammals. The results of GPR54 was in agreement with Han et al. (2020), who found GPR54 was expressed in sertoli cells, leydig cells and spermatids, suggesting the local expression of GPR54 in goats' testes and its autocrine role in leydig cells. Therefore, we concluded that the year-round estrus of rams might be due to high expression level of the GPR54 gene. Of course, further studies are needed to investigate the relationship between KISS1 and GPR54 and ram estrus in more depth.
This study describes the expression pattern of four HPG axis-related genes in year-round estrous (STH) and seasonal estrous (SNT) rams. We found that DIO2 was highly expressed in pituitary, and KISS1 was specifically highly expressed in hypothalamus. The expression levels of EYA3 and GPR54 were high in hypothalamus, pituitary and testis, and the expression levels of the four genes are all higher in STH than SNT. The results suggested that the four genes may regulate the estrous mode of ram via HPG axis, and they may play positive functions in the ram year-round estrus. However, the specific mechanism remains to be further explored. This is the first systematic analysis of tissue expression patterns of the HPG axis-related genes in rams.
The data sets are available upon request from the corresponding author.
These studies were designed by QX and MXC, who performed the experimental analyses and prepared the figures and tables. QX analyzed the data and drafted the manuscript. MXC contributed to revisions of the manuscript. RD, XYH and CHW assisted in interpreting the results and revised the final version of the manuscript. All authors read and approved the final manuscript for publication.
The authors declare that they have no conflict of interest.
We thank Mingxing Chu, Ran Di and all the facilities involved including Chinese Academy of Agricultural Sciences, as well as the local abattoir for their support during this study.
This research has been supported by the National Natural Science Foundation of China (grant nos. 31472078 and 31772580), the Earmarked Fund for China Agriculture Research System (grant no. CARS-38), the Agricultural Science and Technology Innovation Program of China (grant no. ASTIP-IAS13), the China Agricultural Scientific Research Outstanding Talents and Their Innovative Teams Program, China High-level Talents Special Support Plan Scientific and Technological Innovation Leading Talents Program (grant no. W02020274), the Tianjin Agricultural Science and Technology Achievements Transformation and Popularization Program (grant no. 201704020), the Youth Innovative Research and Experimental Project of Tianjin Academy of Agricultural Sciences (grant no. 201915), and the article processing charges were funded by the National Natural Science Foundation of China (grant no. 31472078).
This paper was edited by Steffen Maak and reviewed by two anonymous referees.
An, X. J., Pan, Z. Y., Zhao, S. G., Li, C. Y, Tian, Z. L., Di, R., Liu, Q. Y, Hu, W. P., Wang, X. Y., Zhang, X. S., Zhang, J. L., Cai, Y., and Chu, M. X.: Expression of KiSS-1 and RFRP-3 in Small Tail Han sheep at luteal and follicular periods, Journal of China Agricultural University, 24, 88–96, https://doi.org/10.11841/j.issn.1007-4333.2019.02.09, 2019a.
An, X. J., Zhao, S. G., Pan, Z. Y., Tian, Z. L., Zhang, X. S., Zhang, J. L., Cai, Y., and Chu, M. X.: Tissue expression of DIO2 and DIO3 in Small Tail Han sheep at luteal and follicular periods, Chinese Journal of Animal Science, 55, 73–77, https://doi.org/10.19556/j.0258-7033.2019-01-068, 2019b.
Colledge, W. H.: GPR54 and kisspeptins: Results, Probl. Cell. Differ., 46, 117–143, https://doi.org/10.1007/400_2007_050, 2008.
Dardente, H., Hazlerigg, D. G., and Ebling, F. J. P.: Thyroid hormone and seasonal rhythmicity. Front. Endocrinol (Lausanne), 5, 19, https://doi.org/10.3389/fendo.2014.00019, 2014.
Dardente, H., Lomet, D., Chesneau, D., Pellicer-Rubio, M. T., and Hazlerigg, D.: Discontinuity in the molecular neuroendocrine response to increasing daylengths in Ile-de-France ewes: is transient Dio2 induction a key feature of circannual timing? J. Neuroendocrinol., 31, e12775, https://doi.org/10.1111/jne.12775, 2019.
Dunn, I. C., Wilson, P. W., Shi, Y., Burt, D. W., Loudon, A. S. I., and Sharp, P. J.: Diurnal and photoperiodic changes in thyrotrophin-stimulating hormone β expression and associated regulation of deiodinase enzymes (DIO2, DIO3) in the female juvenile chicken hypothalamus, J. Neuroendocrinol., 29, e12554, https://doi.org/10.1111/jne.12554, 2017.
Dupré, S. M., Miedzinska, K., Duval, C. V., Yu, L., Goodman, R. L., Lincoln, G. A., Davis, J. R. E., Mcneilly, A. S., Burt, D. D., and Loudon, A. S. I.: Identification of Eya3 and TAC1 as long-day signals in the sheep pituitary, Curr. Biol., 20, 829–835, https://doi.org/10.1016/j.cub.2010.02.066, 2010.
Feng, T., Bai, J. H., Xu, X. L., and Liu, Y.: Kisspeptin and its effect on mammalian spermatogensis, Curr. Drug. Metab., 20, 9–14, https://doi.org/10.2174/1389200219666180129112406, 2019.
Funes, S., Hedrick, J. A., Vassileva, G., Markowitz, L., Abbondanzo, S., Golovko, A., Yang, S., Monsma, F. J., and Gustafson, E. L.: The KiSS-1 receptor GPR54 is essential for the development of the murine reproductive system, Biochem. Biophys. Res. Commun., 312, 1357–1363, https://doi.org/10.1016/j.bbrc.2003.11.066, 2003.
Hammond, K. L., Hanson, I. M., Brown, A. G., Lettice, L. A., and Hill, R. E.: Mammalian and Drosophila dachshund genes are related to the Ski proto-oncogene and are expressed in eye and limb, Mech. Dev., 74, 121–131, https://doi.org/10.1016/s0925-4773(98)00071-9, 1998.
Han, Y. G., Zhao, Y. H. T., Si, W. J., Jiang, X. P., Wu, J. Y., Na, R. S., Han, Y. Q., Li, K., Yang, L. G., E,G. X., Zeng, Y., Zhao, Y. J., and Huang, Y. F.: Temporal expression of the KISS1/GPR54 system in goats' testes and epididymides and its spatial expression in pubertal goats, Theriogenology, 152, 114–121, https://doi.org/10.1016/j.theriogenology.2020.04.022, 2020.
Herbison, A. E., Xavier, d. A. d. T., Joanne, D., and Colledge, W. H.: Distribution and postnatal development of Gpr54 gene expression in mouse brain and gonadotropin-releasing hormone neurons, Endocrinology, 151, 312–321, https://doi.org/10.1210/en.2009-0552, 2010.
Huang, D. W., Chu, M. X., Di, R., Liu, Q. Y., Hu, W. P., Wang, X. Y., Pan, Z. Y., and Guo, X. F.: Study on expression of KISS1 and RFRP genes related to reproductive seasonality in goats, Acta Veterinaria et Zootechnica Sinica, 46, 924–931, https://doi.org/10.11843/j.issn.0366-6964.2015.06.007, 2015.
Huang, D. W., Di, R., Hu, W. P., Wang, X. Y., Pan, Z. Y., Guo, X. F., Cao, X. H., Liu, Q. Y., and Chu, M. X.: Expression analysis of DIO2 and DIO3 genes related to reproductive seasonality in goats (Capra hircus), Journal of Agricultural Biotechnology, 24, 1536–1543, https://doi.org/10.3969/j.issn.1674-7968.2016.10.010, 2016.
Ikegami, K. and Yoshimura, T.: Circadian clocks and the measurement of daylength in seasonal reproduction, Mol. Cell. Endocrinol., 349, 76–81, https://doi.org/10.1016/j.mce.2011.06.040, 2012.
Janich, P., Pascual, G., Merlos-Suarez, A., Batlle, E., Ripperger, J., Albrecht, U., Cheng, H. Y. M., Obrietan, K., Croce, L. D., and Benitah, S. A.: The circadian molecular clock creates epidermal stem cell heterogeneity, Nature, 480, 209–214, https://doi.org/10.1038/nature10649, 2011.
Kang, H., Kenealy, T. M., and Cohen, R. E.: The hypothalamic-pituitary-gonadal axis and thyroid hormone regulation interact to influence seasonal breeding in green anole lizards (Anolis carolinensis), Gen. Comp. Endocrinol., 292, 113446, https://doi.org/10.1016/j.ygcen.2020.113446, 2020.
La Y. F., He, X. Y., Zhang, L. P., Di, R., Wang, X. Y., Gan, S. Q., Zhang, X. S., Zhang, J. L., Hu, W. P., and Chu, M. X.: Comprehensive analysis of differentially expressed profiles of mRNA, lncRNA, and circRNA in the uterus of seasonal reproduction sheep, Genes (Basel), 11, 301, https://doi.org/10.3390/genes11030301, 2020.
Li, X., Oghi, K. A., Zhang, J., Krones, A., Bush, K. T., Glass, C. K., Nigam, S. K., Aggarwal, A. K., Maas, R., Rose, D. W., and Rosenfeld, M. G.: Eya protein phosphatase activity regulates Six1-Dach-Eya transcriptional effects in mammalian organogenesis, Nature, 426, 247–254, https://doi.org/10.1038/nature02083, 2003.
Li, S., Ren, J., Yang, G., Guo, Y., and Huang, L.: Characterization of the porcine Kisspeptins receptor gene and evaluation as candidate for timing of puberty in sows, J. Animal. Breed. Genet., 125, 219–227, https://doi.org/10.1111/j.1439-0388.2008.00732.x, 2008.
Lincoln, G.: Melatonin modulation of prolactin and gonadotrophin secretion, Adv. Exp. Med. Biol., 460, 137–153, https://doi.org/10.1007/0-306-46814-x_16, 1999.
Liu, L., Chen, Y., Wang, D., Li, N., Guo, C., and Liu, X.: Cloning and expression characterization in hypothalamic Dio2/3 under a natural photoperiod in the domesticated Brandt's vole (Lasiopodomys brandtii), Gen. Comp. Endocrinol., 259, 45–53, https://doi.org/10.1016/j.ygcen.2017.11.002, 2018.
Livak, K. J.: Analyzing real-time PCR data by the comparative c(t) method, Nat. Protoc., 3, 1101–1108, https://doi.org/10.1038/nprot.2008.73, 2008.
Livak, K. J. and Schmittgen, T. D.: Analysis of relative gene expression data using real-time quantitative PCR and the 2(-delta delta C(T)) method, Methods, 25, 402–408, https://doi.org/10.1006/meth.2001.1262, 2001.
Lomet, D., Cognie, J., Chesneau, D., Dubois, E., Hazlerigg, D., and Dardente, H.: The impact of thyroid hormone in seasonal breeding has a restricted transcriptional signature, Cell. Mol. Life. Sci., 75, 905–919, https://doi.org/10.1007/s00018-017-2667-x, 2018.
Marcheva, B., Ramsey, K. M., Buhr, E. D., Kobayashi, Y., Su, H., Ko, C. H., Ivanova, G., Omura, C., Mo, S., and Vitaterna, M. H.: Disruption of the clock components CLOCK and BMAL1 leads to hypoinsulinaemia and diabetes, Nature, 466, 627–631, https://doi.org/10.1038/nature09253, 2010.
Masumoto, K. H., Ukai-Tadenuma, M.,Kasukawa, T., Nagano, M., Uno, K. D., Tsujino, K., Horikawa, K., Shigeyoshi, Y., and Ueda, H. R.: Acute induction of Eya3 by late-night light stimulation triggers TSHβ expression in photoperiodism, Curr. Biol., 20, 2199–2206, https://doi.org/10.1016/j.cub.2010.11.038, 2010.
Mei, H., Doran, J., Kyle, V., Yeo, S. H., and Colledge, W. H.: Does kisspeptin signaling have a role in the testes?, Front. Endocrinol (Lausanne), 4, 198, https://doi.org/10.3389/fendo.2013.00198, 2013.
Meier, U.: A note on the power of sher's least significant difference procedure, Pharm. Stat., 5, 253–263, https://doi.org/10.1002/pst.210, 2006.
Miao, X. Y., Luo, Q. M., Zhao, H. J., and Qin, X. Y.: Co-expression analysis and identification of fecundity-related long non-coding RNAs in sheep ovaries, Sci. Rep., 6, 39398, https://doi.org/10.1038/srep39398, 2016.
Mishra, I., Bhardwaj, S. K., Malik, S., and Kumar, V.: Concurrent hypothalamic gene expression under acute and chronic long days: Implications for initiation and maintenance of photoperiodic response in migratory songbirds, Mol. Cell. Endocrinol., 439, 81–94, https://doi.org/10.1016/j.mce.2016.10.023, 2017.
Muir, A. I., Chamberlain, L., Elshourbagy, N. A., Michalovich, D., Moore, D. J., Calamari, A., Szekeres, P. G., Sarau, H. M., Chambers, J. K., Murdock, P., Steplewski, K., Shabon, U. Miller, J. E., Middleton, S. E., Darker, J. G., Larminie, C. G., Wilson, S., Bergsma, D. J., Emson, P., Faull, R., Philpott, K. L., and Harrison, D. C.: AXOR12, a novel human G protein-coupled receptor, activated by the peptide KiSS-1, J. Biol. Chem., 276, 28969–28975, https://doi.org/10.1074/jbc.M102743200, 2001.
Nam, D., Yechoor, V. K., and Ma, K.: Molecular clock integration of brown adipose tissue formation and function, Adipocyte, 5, 243–250, https://doi.org/10.1080/21623945.2015.1082015, 2015.
Novaira, H. J., Ng, Y., Wolfe, A., and Radovick, S.: Kisspeptin increases GnRH mRNA expression and secretion in GnRH secreting neuronal cell lines, Mol. Cell. Endocrinol., 311, 126–134, https://doi.org/10.1016/j.mce.2009.06.011, 2009.
Ohtaki, T., Shintani, Y., Honda, S., Matsumoto, H., Hori, A., Kanehashi, K., Kumano, S., Takatsu, Y., Masuda, Y., Ishibashi, Y., Watanabe, T., Asada, M., Yamada, T., Suenaga, M., Kitada, C., Usuki, S., Kurokawa, T., Onda, H., Nishimura, O., and Fujino, M.: Metastasis suppressor gene KiSS-1 encodes peptide ligand of a G-protein-coupled receptor, Nature, 411, 613–617, https://doi.org/10.1038/35079135, 2001.
Oliver, G., Mailhos, A., Wehr, R., Copeland, N. G., and Jenkins, N. A.: Six3, a murine homologue of the sine oculis gene, demarcates the most anterior border of the developing neural plate and is expressed during eye development, Development, 121, 4045–4055, https://doi.org/10.1111/j.1365-2303.1995.tb00491.x, 1996.
Papaoiconomou, E., Msaouel, P., Makri, A., Diamanti-Kandarakis, E., and Koutsilieris, M.: The role of kisspeptin/GPR54 in the reproductive system, Vivo., 25, 343–354, https://doi.org/10.1038/gt.2010.177, 2011.
Park, E., Jung, J., Araki, O., Tsunekawa, K., Park, S. Y., Kim, J., Murakami, M., Jeong, S. Y., and Lee, S.: Concurrent TSHR mutations and DIO2 T92A polymorphism result in abnormal thyroid hormone metabolism, Sci. Rep., 8, 10090, https://doi.org/10.1038/s41598-018-28480-0, 2018.
Popa, S. M., Clifton, D. K., and Steiner, R. A.: The role of kisspeptins and GPR54 in the neuroendocrine regulation of reproduction, Annu. Rev. Physiol., 70, 213–238, https://doi.org/10.1146/annurev.physiol.70.113006.100540, 2008.
Revel, F. G., Saboureau, M., Masson-Pevet, M., Pevet, P., Mikkelsen, J. D., and Simonneaux, V.: Kisspeptin mediates the photoperiodic control of reproduction in hamsters, Curr. Biol., 16, 1730–1735, https://doi.org/10.1016/j.cub.2006.07.025, 2006.
Romano, R. M., Gomes, S. N., Cardoso, N. C. S., Schiessl, L., Romano, M. A., and Oliveira, C. A. New insights for male infertility revealed by alterations in spermatic function and differential testicular expression of thyroid-related genes, Endocrine., 55, 607–617, https://doi.org/10.1007/s12020-016-0952-3, 2017.
Roux, N. D., Genin, E., Carel, J. C., Matsuda, F., Chaussain, J. L., and Milgrom, E.: Hypogonadotropic hypogonadism due to loss of function of the KiSS1-derived peptide receptor GPR54, P. Natl. Acad. Sci. USA, 100, 10972–10976, https://doi.org/10.1073/pnas.1834399100, 2003.
Samir, H., Nagaoka, K., Karen, A., Ahmed, E., El Sayed, M., and Watanabe, G.: Investigation the mRNA expression of KISS1 and localization of kisspeptin in the testes of Shiba goats and its relationship with the puberty and steriodogenic enzymes, Small. Ruminant. Res., 133, 1–6, https://doi.org/10.1016/j.smallrumres.2015.10.024, 2015.
Scarlet D, A. C., Ille, N., Walter, I., Weber, C., Pieler, D., Peinhopf, W., Wohlsein, P., and Aurich, J.: Anti-Muellerian hormone, inhibin A, gonadotropins, and gonadotropin receptors in bull calves after partial scrotal resection, orchidectomy, and Burdizzo castration, Theriogenology, 87, 242–249, https://doi.org/10.1016/j.theriogenology.2016.08.030, 2017.
Seminara, S. B., Messager, S., Chatzidaki, E. E. Thresher, R. R., Acierno Jr, J. S., Shagoury, J. K., Bo-Abbas, Y., Kuohung, W., Schwinof, K. M., Hendrick, A. G., Zahn, D., Dixon, J., Kaiser, U. B., Slaugenhaupt, S. A., Gusella, J. F., Rahilly, S. O., Carlton, M. B. L., Crowley Jr, W. F., Aparicio, S. A. J. R., and Colledge, W. H.: The GPR54 gene as a regulator of puberty, N. Engl. J. Med., 349, 1589–1592, https://doi.org/10.1056/NEJMoa035322, 2003.
Shahed, A. and Young, K. A.: Differential ovarian expression of KiSS-1 and GPR-54 during the estrous cycle and photoperiod induced recrudescence in Siberian hamsters (Phodopus sungorus), Mol. Reprod. Dev., 76, 444–452, https://doi.org/10.1002/mrd.20972, 2009.
Smith, J. T., Cunningham, M. J., Rissman, E. F., Clifton, D. K., and Steiner, R. A.: Regulation of Kiss1 gene expression in the brain of the female mouse, Endocrinology., 146, 3686–3692, https://doi.org/10.1210/en.2005-0488, 2005a.
Smith, J. T., Dungan, H. M., Stoll, E. A., Gottsch, M. L., Braun, R. E, Eacker, S. M., Clifton, D. K., and Steiner, R. A.: Differential regulation of KiSS-1 mRNA expression by sex steroids in the brain of the male mouse, Endocrinology, 146, 2976–2984, https://doi.org/10.1210/en.2005-0323, 2005b.
Tang, J. S., Hu, W. P., Di, R., Liu, Q. Y., Wang, X. Y., Zhang, X. S., Zhang, J. L., and Chu, M. X.: Expression analysis of the prolific candidate genes, BMPR1B, BMP15, and GDF9 in small tail han ewes with three fecundity (FecB gene) genotypes, Animals., 8, 166, https://doi.org/10.3390/ani8100166, 2018.
Tariq, A. R., Shahab, M., Clarke, I. J., Pereira, A., Smith, J. T., Khan, S. H., Sultan, J., Javed, S., and Anwar, T.: Kiss1 and Kiss1 receptor expression in the rhesus monkey testis: a possible local regulator of testicular function, Cent. Eur. J. Biol., 8, 968–974, https://doi.org/10.2478/s11535-013-0219-4, 2013.
Tavolaro, F. M., Thomson, L. M., Ross, A. W., Morgan, P. J., and Helfer, G.: Photoperiodic effects on seasonal physiology, reproductive status and hypothalamic gene expression in young male F344 rats, J. Neuroendocrinol., 27, 79–87, https://doi.org/10.1111/jne.12241, 2014.
Terao, Y., Kumano, S., Takatsu, Y., Hattori, M., Nishimura, A., Ohtaki, T., and Shinrani, Y.: Expression of KiSS-1, a metastasis suppressor gene, in trophoblast giant cells of the rat placenta, Biochim. Biophys. Acta., 1678, 102–110, https://doi.org/10.1016/j.bbaexp.2004.02.005, 2004.
Tomikawa, J., Homma, T., Tajima, S., Shibata, T., Inamoto, Y., Takase, K., Inoue, N., Ohkura, S., Uenoyama, Y., Maeda, K. I., and Tsukamura, H.: Molecular characterization and estrogen regulation of hypothalamic KISS1 gene in the pig, Biol. Reprod., 82, 313–319, https://doi.org/10.1095/biolreprod.109.079863, 2010.
Trivedi, A. K., Sur, S., Sharma, A., Taufique, S. T., and Kumar, V.: Temperature alters the hypothalamic transcription of photoperiod responsive genes in induction of seasonal response in migratory redheaded buntings, Mol. Cell. Endocrinol., 493, 110454, https://doi.org/10.1016/j.mce.2019.110454, 2019.
Xia, Q., Zhang, X. S., Liu, Q. Y., Wang, X. Y., He, X. Y., Guo, X. F., Hu, W. P., Zhang, J. L., Chu, M. X., and Di, R.: Expression patterns of EYA3 and TSHβ in seasonal estrous and year-round estrous sheep, Acta Veterinaria et Zootechnica Sinica, 49, 263–269, https://doi.org/10.11843/j.issn.0366-6964.2018.02.005, 2018.
Xu, P. X., Woo, I., Her, H., Beier, D. R., and Maas, R. L.: Mouse Eya homologues of the Drosophila eyes absent gene require Pax 6 for expression in lens and nasal placode, Development, 124, 219–231, https://doi.org/10.1146/annurev.cellbio.13.1.779, 1997.
Yoshimura, T.: Thyroid hormone and seasonal regulation of reproduction, Front. Neuroendocrinol., 34, 157–166, https://doi.org/10.1016/j.yfrne.2013.04.002, 2013.