the Creative Commons Attribution 4.0 License.
the Creative Commons Attribution 4.0 License.
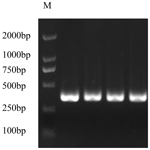
Expression and functional analysis of GnRH at the onset of puberty in sheep
Jihu Zhang
Chenguang Wang
Xiaojun Li
Yongjie Zhang
Feng Xing
Gonadotropin-releasing hormone (GnRH) is a key factor at the onset of puberty. This decapeptide has been found in mammalian ovaries, but its regulatory mechanism in the ovary of sheep at the onset of puberty is not clear. This study investigated the coding sequence (CDS) of the GnRH gene in the ovary of Duolang sheep and the expression of GnRH mRNA in different tissues at the onset of puberty, and analyzed the effect of GnRH on ovarian granulosa cells (GCs) of Duolang sheep. The results showed that the GnRH CDS of sheep was cloned, the full length of the GnRH CDS in sheep ovary was 279 bp, and the nucleotide sequence was completely homologous to that in the hypothalamus. The expression of GnRH mRNA was highest in the hypothalamus and ovary. The expression of related hormones and receptors in GCs of Duolang sheep treated with different concentrations of GnRH for 24 h was affected. GnRH significantly inhibited LH synthesis and LHR expression in GCs. Low concentration (100 ng mL−1) had the most obvious therapeutic effect on follicle-stimulating hormone (FSH) and FSHR. Higher concentration (250 ng mL−1) significantly promoted estradiol and ERβ mRNA. These findings provide strong evidence that ovarian GnRH is an important regulatory factor at the onset of puberty in sheep.
- Article
(3343 KB) - Full-text XML
- BibTeX
- EndNote
In animals puberty is the period of first estrus and ovulation (Spaziani et al., 2020); the animal eventually acquires the ability to reproduce (Witchel et al., 2021). Puberty is considered to be an important marker of the activation of the hypothalamic–pituitary–ovarian axis (HPO) (Smith et al., 2010). Hypothalamic gonadotropin-releasing hormone (GnRH) neurons are the key regulators of puberty and fertility (McIlwraith et al., 2020). At the beginning of puberty, GnRH can activate the established gonad axis (Voliotis et al., 2019). GnRH is secreted from the hypothalamus to the anterior pituitary gland in a pulsating manner during puberty, promoting the synthesis and secretion of follicle-stimulating hormone (FSH) and luteinizing hormone (LH) (Mijiddorj et al., 2017). FSH and LH reach the ovary via blood circulation to promote ovarian development and induce estrogen synthesis in the ovary (Dubois et al., 2002).
At present, the GnRH gene and molecular structure of many species have been elucidated (Gao et al., 2020; Nie et al., 2021), and this decapeptide (pGlu–His–Trp–Ser–Tyr–Gly–Leu–Arg–Pro–Gly–NH2) is a key regulator of reproductive functions (Wu et al., 2019; Flanagan et al., 2017). GnRH in mammals is exactly the same and is formed by removing signal peptide from N-terminal and GnRH-associated peptide (GAP) from C-terminal through enzymatic hydrolysis after synthesis of precursor molecules (Limonta et al., 2018). If the sixth position of the GnRH molecule is replaced with Asp, a agonist with higher activity than natural GnRH can be produced. Conversely, an antagonist that reduces activity is produced by substituting Leu acids with Asp except for the sixth position. Both agonists and antagonists can be used to control reproductive activity in animals. The use of exogenous GnRH will affect not only the regulation of hypothalamic–pituitary processes but also other physiological processes related to reproduction (Zanetti et al., 2020). Khalaf et al. (2010) found that the ovarian granulosa cells (GCs) with the treatment of GnRH antagonist could significantly reduce the content of estradiol (E2). GnRH agonists can affect the expression of gonadotropin receptors in the ovary in prepubertal female cats (Mehl et al., 2017). GnRH and its receptors are expressed in many non-hypothalamic reproductive tissues, such as the ovary, uterus, and fallopian tube (La et al., 2010; Peng et al., 2015). As an important reproductive organ, the ovary plays an important role at the onset of puberty. GnRH can affect ovarian function and participate in related reproductive activities, such as regulating follicular development, steroid synthesis, and ovarian epithelial cell proliferation (Fallah et al., 2020). The GCs are the somatic cells around oocytes in the follicle (Tu et al., 2020), whose proliferation and differentiation directly affect ovarian functional activities such as follicle growth and development, ovulation, luteum formation, and hormone secretion. However, whether ovarian GnRH directly regulates follicular activity is still unknown.
As a representative breed in Xinjiang, China, Duolang sheep (DL) have the advantage of early puberty (Xing et al., 2019). Therefore, this study took Duolang sheep as the research object, cloned the coding sequence (CDS) of the GnRH gene of an ovary, detected the expression of GnRH mRNA in tissues at the onset of puberty, and analyzed the effect of GnRH on GCs in vitro. We also investigated the levels of related hormones (FSH, LH, and E2) and the expression of hormone receptors (GnRHR, LHR, FSHR, ERα, and ERβ) in GCs treated with different concentrations of GnRH.
2.1 Animals and tissue collection
The selected sheep were raised in the Tarim University experimental station (40∘33′2′′ N, 81∘17′4′′ E; altitude 1101 m a.s.l. (above sea level)). Female sheep in prepuberty (145 d age), puberty (within 4–6 h of the first natural estrus), and post-puberty (3 d after the end of estrus) periods were selected. Each period was represented by four sheep, which were of the same strain and reared under the same conditions.
We identified pubertal sheep by detecting changes in estrus and in the vulva. Sheep were deeply anesthetized by intravenous administration of 3 % pentobarbital sodium (Solarbio, Beijing, China) and sacrificed by exsanguination at a healthy physiological stage. After death, the hypothalamus, pituitary gland, ovary, uterus, fallopian tube, heart, liver, spleen, lung, and kidney were removed. After being cut into pieces by sterile surgical scissors, they were placed in cryo-preservation tubes and quickly preserved in liquid nitrogen.
2.2 Culture of granulosa cells from the ovary
Fresh and healthy DL ovaries in puberty were rinsed with 75 % alcohol and then rinsed with phosphate-buffered saline (PBS) three times to completely remove residual alcohol. In Dulbecco's modified eagle medium (DMEM) (Invitrogen, Carlsbad, USA) culture medium, a sterile medical syringe needle was inserted into the follicular cavity of the ovary to absorb the follicular fluid. The follicular fluid and culture medium were mixed and injected into a 15 mL sterile centrifuge tube. The supernatant was discarded after centrifugation at 1000 rpm for 5 min. Following this, 3 mL of DMEM was added, and the mixture was then evenly mixed by light stirring and centrifuged at 1000 rpm for 5 min, after which the supernatant was discarded. The newly prepared and complete DMEM culture medium (10 % fetal bovine serum (FBS) + 1 % double antibody) (Invitrogen, Carlsbad, USA) was added, mixed, and transferred to petri dishes. Samples were placed in an incubator and cultured at 37∘ and 5 % CO2 for 24 h. Next the samples were treated by different concentrations (0, 50, 100, 200, 250 and 500 ng mL−1) of GnRH (gonadorelin, Sansheng, Ningbo, China). After 24 h, the RNA of GCs was extracted, and the supernatant was then used for enzyme-linked immunosorbent assay (ELISA) (LH, E2, and FSH) (Jining, Shanghai, China).
2.3 Total RNA isolation and cDNA synthesis
Trizol (Invitrogen, Carlsbad, USA) was used to extract RNA, and the concentration of extracted RNA was detected using a nucleic acid protein detector (Denovix, Wilmington, USA). The RNA concentration was determined by measuring absorbance at 260 nm. The purity of all RNA was assessed as the ratio of absorbance at 260 and 280 nm (A260 and A280). The cDNA was synthesized using a PrimeScript™ RT reagent kit (TaKaRa, Beijing, China). According to the manufacturer's instructions, the amplification was carried out in a reaction system of 20 µL. The reaction mixture was incubated for 50 min at 42 ∘C, followed by 5 min at 95 ∘C to deactivate the reverse transcriptase.
2.4 Cloning of sheep GnRH gene
The polymerase chain reaction (PCR) was performed in a 25 µL total volume, containing 1 µL of ovarian cDNA, 0.5 µL of each primer (GnRH-1F/R, 10 µM, Table 1), and 12.5 µL of PCR mix (TaKaRa, Beijing, China). Reverse transcription PCR (RT-PCR) with primers (Table 1) was treated as follows: it was treated at 95∘ for 5 min, followed by 35 cycles of 95∘ for 30 s, and annealed for 30 s at 72∘ for 1 min, followed by one cycle at 72∘ for 5 min. The RT-PCR product was ligated with PMD-19T (TaKaRa, Dalian, China). The well-reacted ligands were mixed with DH5α receptor cells (Trans, Beijing, China). The recombinants were selected by a blue-white spot (Solarbio, Beijing, China) reagent and amplified by PCR. The qualified bacterial solution was sent for sequencing.
Table 1Primers used for cloning the CDS of the GnRH gene and qPCR.
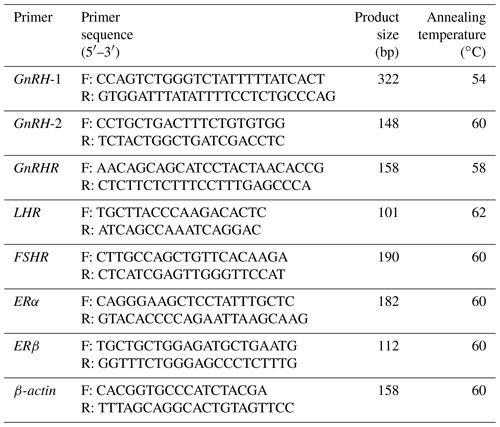
GnRH: XM_015093089.1. GnRHR: NM_ 001009397.1. LHR: NM_001278566.2. FSHR: NM_001009289.1. ERα: XM_042253635.1. ERβ: NM_001009737.1. ACTB (β-actin): NM_001009784.3. Primers are based on the information from NCBI (https://www.ncbi.nlm.nih.gov, last access: 10 September 2021).
2.5 Reverse transcription polymerase chain reaction
The RT-PCR was performed to examine the GnRH expression profile in the hypothalamus, pituitary gland, ovary, fallopian tube, uterus, liver, heart, kidney, lung, and spleen. PCR was performed in a 25 µL total volume solution containing 1 µL of cDNA, 0.5 µL of each primer (10 µM, Table 1), and 12.5 µL of PCR mix (TaKaRa, China). The conditions were the same as cloning. ACTB (β-actin) was used as internal reference, and PCR products were run separately on 1.5 % agarose gels.
2.6 Quantitative real-time PCR
Using cDNA as a template, the expression of GnRH mRNA in hypothalamus, ovary, uterus, and fallopian tube tissue and GnRHR, ERα, ERβ, FSHR, and LHR in GCs were detected using PerfectStart Green qPCR SuperMix (Trans, Beijing, China). Quantitative real-time PCR (qPCR) was performed using 7.5 µL of 2 × Transtant qPCR mix, equimolar amounts of forward and reverse primers (0.5 µL, 10 µM, Table 1), and 1 µL of diluted cDNA (1:2 in RNase-free water) in a final volume of 15 mL. The qPCR was performed under the following conditions: it was treated at 95∘ for 10 min, followed by 40 cycles at 95∘ for 20 s and 60∘ for 30 s and then treated at 95∘ for 15 s, 60∘ for 30 s, and 95∘ for 15 s. Each sample was run three times.
2.7 Statistical analysis
The standard curve was made after the supernatant reproductive hormone concentration was obtained. Cycle threshold (CT) values were calculated using the 2−ΔΔct method (Livak et al., 2001). SPSS 24.0 was used to analyze whether the difference was significant. The difference was considered significant at p<0.05.
3.1 Cloning of CDS of the GnRH gene
The PCR product of the GnRH CDS was detected by 1.5 % agarose gel electrophoresis (Fig. 1). The target fragments of GnRH were consistent with the prediction.
3.2 Similarity comparison and phylogenetic tree construction
DNAMAN 6.0 was used to deduce that the GnRH CDS of Duolang sheep encoded a 92 amino acid protein. The molecular formula was C446H722N124O136S6, and the total molecular weight was 10.19 kDa. ExPASy predicted that the total average hydrophilicity of the protein was −0.218, indicating that the protein was hydrophilic. The isoelectric point was 5.55. The amino acid sequence of GnRH of Duolang sheep was compared with those of a monkey, pig, goat, frog, mouse, and human (Fig. 2). Phylogenetic tree analysis showed that the GnRH of Duolang sheep had the closest relationship with the goat (99 %) and the farthest relationship with the frog (43 %) (Fig. 3).
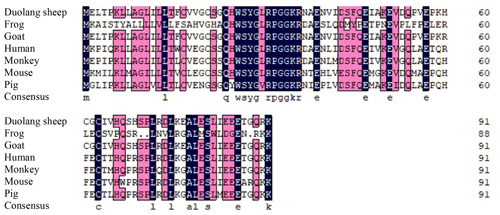
Figure 2Alignment of the amino acid sequences of Duolang sheep GnRH with those of a monkey (NM_001195436.2), pig (NM_001172956.1), goat (XM_013966090.2), frog (XM_ 041587005.1), mouse (NM_008145.3), and human (NM_000825.3).
The secondary structure of the GnRH protein was analyzed by SOPMA software. The secondary structure was mainly composed of α-helix and random coil structures (45.65 % and 39.13 %, respectively), and the rest was made up of extended chain and β-rotation structures (11.96 % and 3.26 %, respectively). A Swiss model was used to predict the tertiary structure of the protein (Fig. 4). This model was established based on template 6F0K.1.g, and the seq similarity was 0.35.
3.3 Expression profile of the GnRH gene in sheep tissues
The expression profiles of GnRH in different tissues at the pubertal stage were assessed (Fig. 5). The results showed that the expression of GnRH was relatively high in the hypothalamus, ovary, and fallopian tube, and weak expression was found in pituitary, uterus, heart, liver, spleen, lung, and kidney tissue.
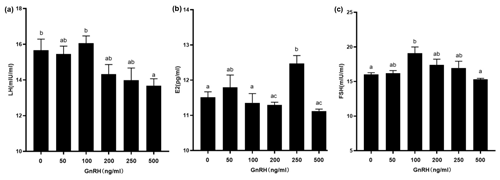
Figure 7Contents of LH, E2, and FSH in GCs at different GnRH concentrations. Bars with different lowercase letters are significantly different at p<0.05, n=3.
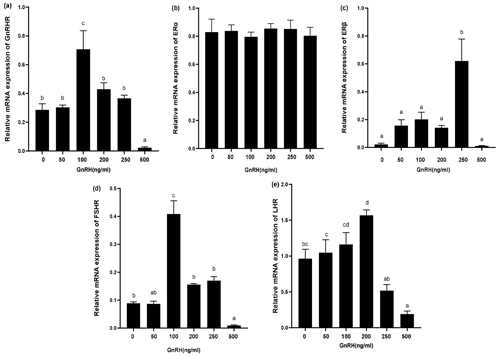
Figure 8Relative mRNA expression of GnRHR, LHR, FSHR, ERα, and ERβ in GCs treated with different GnRH concentrations. Bars with different lowercase letters are significantly different at p<0.05, n=3.
M-DL 2000 markers are as follows: (1) hypothalamus, (2) ovary, (3) pituitary, (4) fallopian tube, (5) uterus, (6) heart, (7) liver, (8) spleen, (9) lung, and (10) kidney.
3.4 The expression of GnRH in tissues at different pubertal stages
In order to study the role of GnRH gene in the reproductive system of Duolang sheep at the onset of puberty, the expression of GnRH mRNA in the hypothalamus, uterus, ovary, and fallopian tube at different pubertal stages was detected by qPCR (Fig. 6). The expression of the hypothalamus was the highest, followed by the ovary and fallopian tube. The expression of GnRH mRNA in the hypothalamus was significantly higher from prepuberty to puberty, and then there was a rapid decline in post-puberty (p<0.05). The expression of GnRH mRNA in ovary was second only to the hypothalamus, which was significantly increased in prepuberty (p<0.05) and significantly decreased in post-puberty (p<0.05). GnRH mRNA was expressed in uterus and fallopian tube, but it was stable in three stages (p>0.05).
Bars with different lowercase letters of the same tissue are significantly different at p<0.05, n=4.
3.5 Effects of exogenous GnRH on LH, E2, and FSH in GCs
In order to study the effects of GnRH on related hormones in ovarian GCs, we treated GCs in puberty of Duolang sheep with different concentrations of GnRH for 24 h (Fig. 7). ELISA showed that high GnRH concentration (500 ng mL−1) significantly inhibited LH (p<0.05). GnRH significantly promoted E2 at 250 ng mL−1 (p<0.05) and promoted FSH at 100 ng mL−1 (p<0.05), but it had no obvious inhibitory effect at high concentration (p>0.05).
3.6 Effects of GnRH on expression of GnRHR, LHR, FSHR, ERα, and ERβ in GCs
The expression of relevant hormone receptors was detected in GCs with different concentrations of GnRH by qPCR (Fig. 8). The results showed that ERα was not affected by GnRH (Fig. 8b). GnRH significantly inhibited GnRHR, FSHR, LHR, and ERβ at high concentrations (500 ng mL−1). Relative mRNA expression of GnRHR was the highest at 100 ng mL−1 and gradually decreased with the increase of GnRH concentration (Fig. 8a). ERβ was most significantly promoted at 250 ng mL−1 (Fig. 8c) and FSHR was most significantly promoted at 100 ng mL−1 (Fig. 8d). GnRH had a significant inhibitory effect on LHR at 500 ng mL−1 (Fig. 8e).
The CDS of GnRH gene in the ovary of Duolang sheep was cloned, and the similarity comparison showed that it had the highest similarity with goat and the lowest similarity with frog. The CDS of the GnRH gene was relatively conserved and was consistent with the characteristics of biological evolution. GnRH gene of sheep consists of three exons and two introns, and the CDS was 279 bp in length, encoding 92 amino acids. Bioinformatics analysis showed that GnRH protein was acidic with an isoelectric point of 5.55. The instability index was 51.75, belonging to unstable protein. The total average hydrophilicity was −0.218, which was a hydrophilic protein, consistent with its transcription pattern. The main secondary structure of the protein was α-helix and random coil, which can ensure the protein generates a more stable tertiary structure, provide enzyme activity sites and other protein-specific functional sites, and generate a specific spatial conformation and tertiary structure.
GnRH was most expressed in the hypothalamus, indicating that it played a key role at the onset of puberty. The combination of GnRH secreted by hypothalamus and GnRHR on pituitary tissue activated the Gq and G11 subfamily of G-protein (Ying et al., 2020) and ultimately promotes the synthesis and release of gonadotropin, thus initiating the puberty. Due to the rapid decomposition of GnRH in the body and its massive dilution in blood circulation, the role of GnRH in the hypothalamus is limited to the pituitary tissue (Litichever et al., 2009). Therefore, the reproductive function of GnRH outside the hypothalamus has attracted special attention in recent years. We studied GnRH mRNA in 10 types of tissue from Duolang sheep, indicating that it can function according to the type of target tissues and physiological conditions. The relative mRNA expression of GnRH in the ovary of Duolang sheep was second only to that in the hypothalamus, and the expression trend was consistent with that in the hypothalamus, suggesting ovarian GnRH also played an important role at the onset of puberty. GnRH mRNA in the ovaries of ducks was significantly increased from prepuberty to puberty (Ni et al., 2007). In situ hybridization revealed the localization of GnRH mRNA in primary, secondary, and tertiary GCs (Clayton et al., 1992; Whitelaw et al., 1995). The rapid decrease of GnRH mRNA in ovary from puberty to post-puberty indicated that GnRH was related to luteal formation after ovulation. Choi et al. (2006) also found that GnRH and GnRHR were strongly expressed in human corpus luteum granulosa cells. These studies suggest that GnRH is an important autocrine and paracrine regulator in the ovary.
It has been confirmed that GnRH and its receptor exist in animal ovaries and play important physiological roles in animal reproductive cycle (Schirman-Hildesheim et al., 2005; Singh et al., 2008). In the ovary, GnRH needs to bind to GnRHR to work. A common feature of GnRHR in mammals is the absence of the carboxy terminal tail present in all other receptors in the G-protein-coupled receptors family (Blomenrohr et al., 1999; Vrecl et al., 2000), resulting in slow receptor internalization and rapid desensitization (Metallinou et al., 2007). The expression of GnRHR was the highest when GnRH was 100 ng mL−1. With the increase in concentration, the expression of GnRHR decreased gradually, indicating that high concentrations of GnRH can inhibit the expression of its receptor and that desensitization may also exist. GnRH regulates the synthesis of steroids, such as estrogen, and also affects ovarian gonadotropin production. In mouse ovaries, low doses of GnRH promoted ovarian steroid production and follicular development and high doses of GnRH inhibited follicular development and ovulation (Singh et al., 2010). It is also associated with the transformation of some genes in follicular maturation and ovulation, such as tissue fibrinogen. GCs with the treatment of GnRH significantly increased the levels of FSH and E2, indicating that GnRH can promote the expression of ovarian FSH and E2. GnRH mRNA was high in the ovary in prepuberty, possibly to stimulate the expression of FSH and E2 to promote follicular development. It was obvious that the GnRH had no significant effect on ERα expression, while ERβ changed with the concentration change. Follicles of mice lacking ERα still matured and were still able to ovulate (Byers et al., 1997; Couse et al., 1999). Surprisingly, ERβ-deficient mice failed to develop follicles and ovulate (Jayes et al., 2014). These studies suggested that the main receptor of E2 in follicles was ERβ. GCs are the main locus of ERβ regulation gene expression, which expresses ERβ and is involved in the production of steroids and gonadotropins, and ERβ is essential for follicular development and ovulation (Lee et al., 2021). However, it is not known whether the increase in ERβ was caused directly by the exogenous GnRH or the E2 caused by GnRH. E2 and ERβ production were the highest in GCs at high concentrations (250 ng mL−1) of GnRH. GnRH can promote the formation of ERβ and E2 and promote follicular development in prepuberty. GnRH increased significantly in puberty and increased E2 and ERβ expression, which may be related to ovulation. FSH is essential for the ovary to perform its reproductive function. Li et al. (2019) found that FSHR was expressed in the follicular stage and luteal stage in two different breeds of sheep in China. GCs have receptor sites for many other hormonal and non-hormonal factors through which FSH can exert stimulatory or inhibitory effects. FSH can activate camp-dependent physiological processes, promote the activity of steroid hormone-producing enzymes in ovarian granulosa cells, and increase E2 in blood. FSH and E2 can jointly promote the proliferation of GCs, the enlargement of follicular cavity, and the development of follicles. The inhibition of LH and LHR was obvious at high GnRH concentration (500 ng mL−1). GnRH agonist can significantly inhibit LH expression in rat ovarian GCs (Litichever et al., 2009). GnRH inhibited ovarian LHR expression in cats in puberty (Mehl et al., 2017). These findings suggest that ovarian GnRH plays a role by down-regulating LH and LHR. The content of GnRH mRNA in the ovary was lower from puberty to post-puberty, which may be related to the formation of luteum.
In general, GnRH CDS of Duolang sheep had the highest similarity with goat. The expression of GnRH in the hypothalamus and ovary was the highest in puberty. In ovarian GCs, GnRH significantly inhibited LH and LHR. Moderate GnRH can up-regulate FSH, E2, FSHR, and ERβ. These results suggested that ovarian GnRH was involved at the onset of puberty in sheep and may be related to ovarian follicular development and ovulation.
The original data are available upon request to the corresponding authors.
JZ participated in the design of the study, conducted the analysis, and was the primary author of the paper. FX participated in the design of the study. CW and XL participated in data analysis and drafting the paper. YZ participated in the design of the study and drafted and reviewed the paper. All authors read and approved the final paper.
The contact author has declared that none of the authors has any competing interests.
This study was conducted in accordance with the specifications of the Ethics Committee of Tarim University of Science and Technology (SYXK 2020-009).
Publisher's note: Copernicus Publications remains neutral with regard to jurisdictional claims in published maps and institutional affiliations.
We thank all the facilities involved, including Tarim University and Xinjiang Production and Construction Corps.
This research has been supported by the Foundation for Innovative Research Groups of the National Natural Science Foundation of China (grant no. 31660652).
This paper was edited by Joachim Weitzel and reviewed by two anonymous referees.
Blomenrohr, M., Heding, A., Sellar, R., Leurs, R., Bogerd, J., Eidne, K. A., and Willars, G. B.: Pivotal role for the cytoplasmic carboxyl-terminal tail of a nonmammalian gonadotropin-releasing hormone receptor in cell surface expression, ligand binding, and receptor phosphorylation and internalization, Mol. Pharmacol., 56, 1229–1237, https://doi.org/10.1124/mol.56.6.1229, 1999.
Byers, M., Kuiper, G. G., Gustafsson, J. A., and Park-Sarge, O. K.: Estrogen receptor-beta mRNA expression in rat ovary: down-regulation by gonadotropins, Mol. Endocrinol., 11, 172–182, https://doi.org/10.1210/mend.11.2.9887, 1997.
Choi, J., Gilks, C. B., Auersperg, N., and Leung, P. C. K.: Immunolocalization of gonadotropin-releasing hormone (GnRH)-I, GnRH-II, and type I GnRH receptor during follicular development in the human ovary, J. Clin. Endocrinol. Metab. 91, 4562–4570, https://doi.org/10.1210/jc.2006-1147, 2006.
Clayton, R. N., Eccleston, L., Gossard, F., and Morel, G.: Rat granulosa cells express the gonadotropin-releasing hormone gene: evidence from in situ hybridisation, J. Mol. Endocrinol., 9, 189–195, https://doi.org/10.1677/jme.0.0090189, 1992.
Couse, J. F. and Korach, K. S.: Reproductive phenotypes in the estrogen receptor-alpha knockout mouse, Ann. Endocrinol., 60, 143–148, https://doi.org/10.1016/S0303-7207(99)00036-2, 1999.
Dubois, E. A., Zandbergen, M. A., Peute, J., and Goos, H. J.: Evolutionary development of three gonadotropin-releasing hormone (GnRH) systems in vertebrates, Brain. Res. Bull., 57, 413–418, https://doi.org/10.1016/s0361-9230(01)00676-1, 2002.
Fallah, H. P. and Habibi, H. R.: Role of GnRH and GnIH in paracrine/autocrine control of final oocyte maturation, Gen. Comp. Endocrinol., 299, 113619, https://doi.org/10.1016/j.ygcen.2020.113619, 2020.
Flanagan, C. A. and Manilall, A.: Gonadotropin-Releasing Hormone (GnRH) Receptor Structure and GnRH Binding, Front Endocrinol., 8, 274, https://doi.org/10.3389/fendo.2017.00274, 2017.
Gao, G., Zhang, K., Zhao, X., Wu, R., Zhong, H., Li, J., Li, C., Xie, Y., and Wang, Q.: Molecular cloning of the goose GnRH gene and identification of GnRH polymorphisms associated with laying traits, Br. Poult. Sci., 61, 502–507, 2020.
Jayes, F. L., Burns, A. K., Rodriguez, K. F., Kissling, G. E., and Korach, K. S.: The Naturally Occurring Luteinizing Hormone Surge Is Diminished in Mice Lacking Estrogen Receptor Beta in the Ovary, Biol. Reprod., 90, 1–9, https://doi.org/10.1095/biolreprod.113.113316, 2014.
Khalaf, M., Mittre, H., Levallet, J., Hanoux, V., Denoual, C., Herlicoviez, M., Bonnamy P. J., and Benhaim, A.: GnRH agonist and GnRH antagonist protocols in ovarian stimulation: differential regulation pathway of aromatase expression in human granulosa cells, Reprod. Biomed., 21, 56–65, https://doi.org/10.1016/j.rbmo.2010.03.017, 2010.
La, Y., He, X., Zhang, L., Di, R., Wang, X., Gan, S., Zhang, X., Zhang, J., Hu. W., and Chu, M.: Comprehensive Analysis of Differentially Expressed Profiles of mRNA, lncRNA, and circRNA in the Uterus of Seasonal Reproduction Sheep, Genes, 11, 19, https://doi.org/10.3390/genes11030301, 2020.
Lee, E. B., Chakravarthi, V. P., Wolfe, M. W., and Rumi, M. A. K.: ERβ Regulation of Gonadotropin Responses during Folliculogenesis, Int. J. Mol. Sci., 22, 10348, https://doi.org/10.3390/ijms221910348, 2021.
Li, L., Lu, S., Ma, Q., Wan, P., Liu, C., Yang, H., Yang, H., Yang, Y., Dai, R., and Shi, G.: The comparison of reproductive hormone receptor expressions of the sheep ovary and hormone concentrations in two Chinese breeds, Reprod. Domest. Anim., 54, 892–901, https://doi.org/10.1111/rda.13441, 2019.
Limonta, P., Marelli, M., Moretti, R., Marzagalli, M., Fontana, F., and Maggi, R.: GnRH in the Human Female Reproductive Axis, Vitam. Horm., 107, 27–66, https://doi.org/10.1016/bs.vh.2018.01.003, 2018.
Litichever, N., Gershon, E., Dekel, N., and Koch, Y.: Hormonal Regulation of GnRH and LHβ mRNA Expression in Cultured Rat Granulosa Cells, J. Mol. Neurosci., 39, 78–85, https://doi.org/10.1007/s12031-009-9185-8, 2009.
Livak, K. J. and Schmittgen, T. D.: Analysis of Relative Gene Expression Data using Real-Time Quantitative PCR, Methods, 25, 402–408, https://doi.org/10.1006/meth.2001.1262, 2001.
McIlwraith, E. K. and Belsham, D. D.: Hypothalamic reproductive neurons communicate through signal transduction to control reproduction, Mol. Cell Endocrinol., 518, 110971, https://doi.org/10.1016/j.mce.2020.110971, 2020.
Mehl, N. S., Srisuwatanasagul, S., Swangchan-Uthai, T., Sirivaidyapong, S., and Khalid, M.: GnRH-agonist implants suppress reproductive function and affects ovarian LHR and FSHR expression in prepubertal female cats, Theriogenology, 87, 250–258, https://doi.org/10.1016/j.theriogenology.2016.09.003, 2017.
Metallinou, C., Asimakopoulos, B., Schröer, A., and Nikolettos, N.: Gonadotropin-Releasing Hormone in the Ovary, Reprod. Sci., 14, 737–749, https://doi.org/10.1177/1933719107310707, 2007.
Mijiddorj. T., Kanasaki, H., Sukhbaatar, U., Oride, A., Hara, T., and Kyo, S.: Mutual regulation by GnRH and kisspeptin of their receptor expression and its impact on the gene expression of gonadotropin subunits, Gen. Comp Endocrinol., 246, 382–389, https://doi.org/10.1016/j.ygcen.2017.01.014, 2017.
Ni, Y., Zhou, Y., Lu, L., Grossmann, R., and Zhao, R.: Developmental changes of FSH-R, LH-R, ER-beta and GnRH-I expression in the ovary of prepubertal ducks (Anas platyrhynchos), Anim. Reprod. Sci., 100, 318–328, https://doi.org/10.1016/j.anireprosci.2006.08.012, 2007.
Nie, H., Zhang, R., Yu, X., Zhang, Y., Yan, P., Li, E., Wang, R., and Wu, X.: Molecular cloning, immunological characterization, and expression analysis of gonadotropin-releasing hormone (GnRH) in the brain of the Chinese alligator during different stages of reproductive cycle, Gene, 789, 145672, https://doi.org/10.1016/j.gene.2021.145672, 2021.
Peng, B., Klausen, C., Campbell, L., Leung, P. C. K., Horne, A. W., and Bedaiwy, M. A.: Gonadotropin-releasing hormone and gonadotropin-releasing hormone receptor are expressed at tubal ectopic pregnancy implantation sites, Fertil. Steril., 105, 1620–1627, https://doi.org/10.1016/j.fertnstert.2016.02.003, 2015.
Schirman-Hildesheim, T. D., Bar, T., Ben-Aroya, N., and Koch, Y.: Differential gonadotropin-releasing hormone (GnRH) and GnRH receptor messenger ribonucleic acid expression patterns in different tissues of the female rat across the estrous cycle, Endocrinology, 146, 3401–3408, https://doi.org/10.1210/en.2005-0240, 2005.
Singh, P., Krishna, A., Sridaran, R., and Tsutsui, K.: Changes in GnRH I, bradykinin and their receptors and GnIH in the ovary of Calotes versicolor during reproductive cycle, Gen. Comp. Endocrinol., 159, 158–69, https://doi.org/10.1016/j.ygcen.2008.08.016, 2008.
Singh, P. and Krishna, A.: Effects of GnRH agonist treatment on steroidogenesis and folliculogenesis in the ovary of cyclic mice, J. Ovarian Res., 3, 26, https://doi.org/10.1186/1757-2215-3-26, 2010.
Smith, J. T. and Clarke, I. J.: Seasonal breeding as a neuroendocrine model for puberty in sheep, Mol. Cell Endocrinol., 324, 102–109, https://doi.org/10.1016/j.mce.2010.03.007, 2010.
Spaziani, M., Tarantino, C., Tahani, N., Gianfrilli, D., Sbardella, E., Lenzi, A., and Radicioni, A. F.: Hypothalamo-Pituitary axis and puberty, Mol. Cell Endocrinol., 520, 111094, https://doi.org/10.1016/j.mce.2020.111094, 2020.
Tu, J., Chen, Y., Li, Z., Yang, H., Chen, H., and Yu, Z.: Long non-coding RNAs in ovarian granulosa cells, J. Ovarian Res. 13, 63, https://doi.org/10.1186/s13048-020-00663-2, 2020.
Voliotis, M., Li, X. F., De, B. R., Lass, G., Lightman, S. L, O'Byrne, K. T., and Tsaneva-Atanasova, K.: The Origin of GnRH Pulse Generation: An Integrative Mathematical-Experimental Approach, J. Neurosci., 39, 9738–9747, https://doi.org/10.1523/JNEUROSCI.0828-19.2019, 2019.
Vrecl, M., Heding, A., Hanyaloglu, A., Taylor, P. L., and Eidne, K. A.: Internalization kinetics of the gonadotropin-releasing hormone (GnRH) receptor, Pflugers. Arch., 439, r019–r020, https://doi.org/10.1007/s004240000075, 2000.
Whitelaw, P. F., Eidne, K. A., Sellar, R., Smyth, C. D., and Hillier, S. G.: Gonadotropin-releasing hormone receptor ribonucleic acid expression in rat ovary, Endocrinology, 136, 172–179, https://doi.org/10.1210/endo.136.1.7828528, 1995.
Witchel, S. F. and Plant. M. T.: Neurobiology of puberty and its disorders, Handb. Clin. Neurol., 181, 463–496, https://doi.org/10.1016/B978-0-12-820683-6.00033-6, 2021.
Wu, H. M., Wang, H. S., Huang, H. Y., Soong, Y. K., MacCalman, C. D., and Leung, P. C.: GnRH signaling in intrauterine tissues, Reproduction, 137, 769–77, https://doi.org/10.1530/REP-08-0397, 2009.
Xing, F., Zhang, C., and Kong, Z.: Cloning and expression of lin-28 homolog B gene in the onset of puberty in Duolang sheep, Asian-Austral. J. Anim. Sci., 32, 23–30, https://doi.org/10.5713/ajas.18.0276, 2019.
Ying, S., Qin, J., Dai, Z., An, H., Zhu, H., Chen, R., Yang, X., Wu, W., and Shi, Z.: Effects of LPS on the Secretion of Gonadotrophin Hormones and Expression of Genes in the Hypothalamus-Pituitary-Ovary (HPG) Axis in Laying Yangzhou Geese, Animals, 10, 2259–2270, https://doi.org/10.3390/ani10122259, 2020.
Zanetti, B. F., Braga, D. P. A. F., Setti, A. S., Iaconelli, A., and Borges, E.: Effect of GnRH analogues for pituitary suppression on oocyte morphology in repeated ovarian stimulation cycles, JBRA Assist. Reprod., 24, 24–29, https://doi.org/10.5935/1518-0557.20190050, 2020.